An unhealthy diet: making sense of a fatty niche for leukemia stem cells
In an era of obesity epidemics and surging cardiovascular mortality, new evidence has linked our unhealthy diets with an unexpected danger—cancer cell survival and resistance to chemotherapy. Leukemia stem cells (LSCs) are the prototypical cancer stem cell, defined by their ability to initiate and maintain leukemia and also to differentiate into mature progeny that lack this self-renewal ability (1). Analogous to hematopoietic stem cells (HSC), their counterparts in normal development, it has been postulated that LSCs also coopt or hijack cell extrinsic support from secreted or cellular factors to support their own survival within the bone marrow and other hematopoietic niches (2,3). Crosstalk between LSCs and extra-cellular supports may provide an important microenvironment enriched in specific factors favoring LSCs quiescence, survival and chemo-resistance. For example, LSCs home to and engraft endosteal surfaces and these endosteal LSCs appear resistant to the effects of standard chemotherapy (4). LSC homing is dependent on surface receptors such as CD44 (5,6) and may also be regulated by cell intrinsic pathways of self-renewal within LSCs (7) (see Figure 1). A greater understanding how LSCs interact within their potentials niches may lead to novel targeted therapies that potently synergize with chemotherapy and improve survival of patients with leukemia (8).
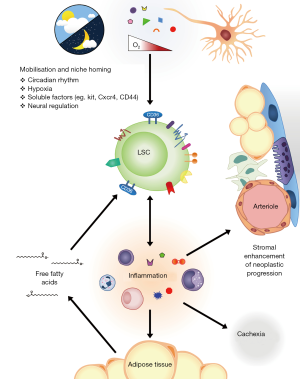
A recent report in Cell Stem Cell by Drs Ye, Jordan and colleagues (9) provides novel and provocative insights into the interaction between LSCs and a hitherto unappreciated cancer stem cell niche, adipose tissue (AT). Using well-defined retroviral models of murine blast crisis chronic-myeloid leukemia (bcCML) and acute myeloid leukemia (AML), they identify enrichment of LSCs numbers within murine gonadal adipose tissue (GAT) and demonstrate preserved LSC function within GAT LSCs. These GAT LSCs were enriched for a fatty acid transporter, CD36, and CD36+ LSCs retained LSC function in stringent transplantation assays. These CD36+ LSCs were also enriched for quiescent and chemoresistant cells, raising the tantalizing observation that this GAT may be a niche that promotes the survival and chemoresistance of LSCs in vivo.
Why then would LSCs be drawn towards fatty niche cells? Obesity and adipose tissue is a source of chronic inflammation that in turn can impact many stages of tumorigenesis including tumor initiation [e.g., IL-1α can stimulate stromal cell and potentially stimulated neoplastic progression (10)] and through cytokine and growth factor signaling (11). In this paper, Ye et al. demonstrate functionally active CD36 expression on the LSC surface and this expression was required for the adipocyte-proximal localization of LSCs although homing to bone marrow was independent of CD36. Corresponding with this, the authors showed that LSCs from AT have a proinflammatory gene expression program and actually produce proinflammatory cytokines, leading to free fatty acid release and atrophy from AT leading to further inflammation. This finding also correlated with lipolytic characteristics of the LSCs and the development of cancer cachexia within mice, mediated by systemic factors, which lead to weight loss, even in low leukemic burden tissues. LSCs could sustain lipolysis by production of inflammatory cytokines such as IL-1α, IL-1β, TNF-α and CSF2. Moreover, the release of fatty acids mediates fatty acid oxidation, which may in turn orchestrate LSCs stemness and survival (12).
HSCs need to maintain quiescence for their normal function (13) and to do this, they exhibit a specific metabolic profile characterized by weak mitochondrial activity, low ATP level and are highly dependent on glycolysis. Low mitochondrial activity prevents reactive oxygen species (ROS) production and thus differentiation of HSCs. It appears that fatty acid oxidation has a crucial role in HSCs maintenance and in the regulation of asymmetric versus symmetric differentiation, mediated primarily through lipid activated transcription factors from peroxysome proliferator activated receptor family (PPARs) (12,14). In consonance with this finding, inhibition of PPARδ has been shown to promote HSCs exhaustion. Intriguingly, PPARγ inhibition also leads to erosion of LSCs bulk by pushing them out of quiescence in the context of CML (15). It appears that this metabolic pathway may target LSCs preferentially over HSCs, again suggesting that lipid metabolism is more critical for LSCs quiescence maintenance.
Altogether, Ye et al. provide new evidence on microenvironment importance in the biology of LSCs in a novel, extramedullary niche. This study provides a new link implicating the interplay between LSCs and adipose tissue for disease drug resistance and clinical outcome. However, there are a number of important, and unanswered questions regarding lipid metabolism in LSCs. Firstly, fat distribution is different in these murine models compared to patients with leukaemia. Are such LSC reservoirs found in obese, or non-obese, patients with AML and are these reservoirs conserved between species? How is surface CD36 regulated by oncogenes and within subtypes of leukaemia? What are the toxicities of targeting fatty acid metabolism alone, and in combination with chemotherapy, and how might this lead to interaction with other established supportive care protocols such as broad-spectrum antifungal, antiviral and antibacterial therapies?
These provocative findings lead us to the inevitable question of how to translate this fascinating finding into meaningful improvements for patients suffering from AML. It is unlikely that simply targeting CD36 with a monoclonal antibody, or even an agent to alter fatty acid transport, will be sufficient to prevent leukemia progression or relapse. CD36 deficient leukaemia cells were still capable of giving rise to AML, even though this was in anatomically distinct, classical haematopoietic niches. Furthermore, the highly catabolic state of overt leukemia and the inevitable neutropenic fevers that patients endure during induction chemotherapy is not an appropriate time to intervene with caloric restriction. Perhaps the opportunity for specific LSC targeting, including via alterations of metabolic profile, resides within the tentative status of clinical remission with persistent minimal residual disease (16). More evidence is still needed to correlate CD36 and lipid biosynthesis with aggressive leukaemia and clinical resistance to chemotherapy (i.e., relapse after treatment) prior to pursuing these approaches in a prospective clinical trial.
Acknowledgments
Funding: SWL gratefully acknowledges the support of the Gordon and Jessie Gilmour Trust.
Footnote
Provenance and Peer Review: This article was commissioned and reviewed by the Section Editor Lichao Sun (State Key Laboratory of Molecular Oncology, National Cancer Center (NCC)/Cancer Hospital, Chinese Academy of Medical Sciences (CAMS), Peking Union Medical College, Beijing, China).
Conflicts of Interest: SWL has received funding from Cancer Australia, the National Health and Medical Research Council of Australia and the Leukaemia Foundation. The other authors have no conflicts of interest to declare
Ethical Statement: The authors are accountable for all aspects of the work in ensuring that questions related to the accuracy or integrity of any part of the work are appropriately investigated and resolved.
Open Access Statement: This is an Open Access article distributed in accordance with the Creative Commons Attribution-NonCommercial-NoDerivs 4.0 International License (CC BY-NC-ND 4.0), which permits the non-commercial replication and distribution of the article with the strict proviso that no changes or edits are made and the original work is properly cited (including links to both the formal publication through the relevant DOI and the license). See: https://creativecommons.org/licenses/by-nc-nd/4.0/.
References
- Lane SW, Gilliland DG. Leukemia stem cells. Semin Cancer Biol 2010;20:71-6. [Crossref] [PubMed]
- Lane SW, Scadden DT, Gilliland DG. The leukemic stem cell niche: current concepts and therapeutic opportunities. Blood 2009;114:1150-7. [Crossref] [PubMed]
- Lane SW, Williams DA, Watt FM. Modulating the stem cell niche for tissue regeneration. Nat Biotechnol 2014;32:795-803. [Crossref] [PubMed]
- Ishikawa F, Yoshida S, Saito Y, et al. Chemotherapy-resistant human AML stem cells home to and engraft within the bone-marrow endosteal region. Nat Biotechnol 2007;25:1315-21. [Crossref] [PubMed]
- Krause DS, Lazarides K, Lewis JB, et al. Selectins and their ligands are required for homing and engraftment of BCR-ABL1+ leukemic stem cells in the bone marrow niche. Blood 2014;123:1361-71. [Crossref] [PubMed]
- Krause DS, Lazarides K, von Andrian UH, et al. Requirement for CD44 in homing and engraftment of BCR-ABL-expressing leukemic stem cells. Nat Med 2006;12:1175-80. [Crossref] [PubMed]
- Lane SW, Wang YJ, Lo Celso C, et al. Differential niche and Wnt requirements during acute myeloid leukemia progression. Blood 2011;118:2849-56. [Crossref] [PubMed]
- Nervi B, Ramirez P, Rettig MP, et al. Chemosensitization of acute myeloid leukemia (AML) following mobilization by the CXCR4 antagonist AMD3100. Blood 2009;113:6206-14. [Crossref] [PubMed]
- Ye H, Adane B, Khan N, et al. Leukemic Stem Cells Evade Chemotherapy by Metabolic Adaptation to an Adipose Tissue Niche. Cell Stem Cell 2016;19:23-37. [Crossref] [PubMed]
- Grivennikov SI, Greten FR, Karin M. Immunity, inflammation, and cancer. Cell 2010;140:883-99. [Crossref] [PubMed]
- Mantovani A, Allavena P, Sica A, et al. Cancer-related inflammation. Nature 2008;454:436-44. [Crossref] [PubMed]
- Ito K, Carracedo A, Weiss D, et al. A PML–PPAR-δ pathway for fatty acid oxidation regulates hematopoietic stem cell maintenance. Nat Med 2012;18:1350-8. [Crossref] [PubMed]
- Walter D, Lier A, Geiselhart A, et al. Exit from dormancy provokes DNA-damage-induced attrition in haematopoietic stem cells. Nature 2015;520:549-52. [Crossref] [PubMed]
- Ito K, Bernardi R, Morotti A, et al. PML targeting eradicates quiescent leukaemia-initiating cells. Nature 2008;453:1072-8. [Crossref] [PubMed]
- Prost S, Relouzat F, Spentchian M, et al. Erosion of the chronic myeloid leukaemia stem cell pool by PPARγ agonists. Nature 2015;525:380-3. [Crossref] [PubMed]
- Ivey A, Hills RK, Simpson MA, et al. Assessment of Minimal Residual Disease in Standard-Risk AML. N Engl J Med 2016;374:422-33. [Crossref] [PubMed]