Potential and importance of metalloproteinases and interleukins in inflammation and metastasization in non-small cell lung cancer
Introduction
The relation between cancer and the immune system has been studied over the years. In the past, it was observed that some tumours were infiltrated by many cells of the immune system and that those infiltrates were supposedly related to the attempt, by the host immune system, to eradicate the tumour (1). Nowadays, it is clear that the tumour associated inflammation has a paradoxical effect of enhancing carcinogenesis, and its progression is considered as an enabling characteristic of cancer (1). However, immunosurveillance is also present and the immune system acts against transformed cells, but eventually that combat interaction fades and components of the immune system are also capable of hosting an immune microenvironment that anchors the tumour and gives room for it to grow and to develop (1-5).
Lung cancer continues to be the deadliest cancer of all and non-small cell lung cancer (NSCLC) represents the majority of cases (6). There are many genetic alterations associated and many risk factors, such as the tobacco smoke (6-16). Nowadays, precise and personalised medicine has become top priority. The discovery of the loss of standard biomarkers allows us, today, to have more targeted therapies and immunotherapies that enhance our options fighting this cancer (17-19). It becomes crucial to approach the right patient, at the right time, with the right drug, dose and schedule (18). However, drug resistance still remains an obstacle to surpass that limits the effectiveness of targeted therapies. Therefore, the finding of new biomarkers not only can be used as part of new therapies, but also allow us to understand and evaluate which patients can benefit from which therapy (20,21).
In this review, the role of some biomolecules will be explored. Special attention will be paid to the metalloproteinases (MMPs), the tissue inhibitors of metalloproteinases (TIMPs) and the interleukins (ILs). These markers of inflammation and metastasization play important roles in carcinogenesis, given that the MMPs and the TIMPs have already been associated with metastasization, angiogenesis or tumour growth; while ILs are important intermediates of our immune system and have already been associated with inflammation and carcinogenesis (22,23). Due to their relationship with carcinogenesis and metastasization as well as with inflammation, their potential to be used in clinical practice as prognosis or treatment response markers remains possible. Hence, this review will also consider not only what these biomolecules are, but also their importance and function in the NSCLC.
Immune system, tumour microenvironment and NSCLC
The tumour development is usually associated with inflammation which can become a chronic condition later on (2). The immune system escape is a fundamental hallmark of cancer and the interaction between the tumour and the immune system suggests that there is an elimination of the nascent tumour cells by the immune system, followed by an equilibrium stage where the immune system reaction can control the tumour progression, and finally by the escape stage when tumour cells are able to resist the immune system effect by immunoediting and evading immune system response (2-4). During lung cancer progression, the tumour cells interact with the host and create the microenvironment where local cancer-antigen-specific immune system response is shaped (5). Some elements of the immune system acquire changes that enable the tumour to avoid destruction and those elements may play a role in future targeted therapies or immunotherapies (5).
In NSCLC, besides an infiltration of different types of immune system cells, there is also an expression of inflammatory molecules that are normally related to tumour progression, evasion of the immune system response, invasion and metastasis (2), as well as an expression of immune inhibitory molecules in the tumour microenvironment (17). A feature of almost all cancers and their microenvironments is the leukocyte infiltration, which includes mast cells, Natural Killer (NK) cells, T and B lymphocytes and, in particular, the tumour-associated macrophages (TAMs) (2). These infiltrates are associated with angiogenesis and other processes that can be prejudicial to the host and that are essential for the tumour microenvironment to endure and for the tumour to progress (2).
Macrophages are one of the most important immune system cells in the immune and tumour microenvironment. There are two pathways for the macrophages to differentiate, the M1 or the M2 activation (24). When macrophages go into M1 differentiation they become effector cells, whereas M2 macrophages are capable of suppressing the immune system response, promote tissue remodelling and angiogenesis (24). These M2 macrophages are usually present in the tumour microenvironment, indicating an immune suppressing environment (24). In the acute response, macrophages, dendritic cells and mast cells are effectors capable of mediating this response by releasing cytokines, reactive oxygen species and other mediators (2,25). Direct T-cell recognition of tumour cells requires the presence of antigenic peptides, usually presented by major histocompatibility complex (MHC) molecules (17). In a significant number of lung cancer cases, there is a deregulation of components, which are part of this machinery, via genetic and epigenetic alterations, leading cancer cells to avoid death by the T cell activity (17). Suppressed presenting antigen molecules are one of the mechanisms that lead to the immune response escape and, in some cases, it can be reversed by interferon-γ (IFN-γ) (26). This is important in the immunotherapy context, as there is a possibility that NK cells and T cells can be activated within the tumour microenvironment and as they are a source of IFN-γ (17). According to some studies, cancer cells can also evade the immune system response by immunoediting and inducting antigen-specific tolerance (27,28). The immunosurveillance in lung cancer is effective in early stages of carcinogenesis, but eventually gets inhibited and the tumour progresses (29). The low antigenicity of lung cancer cells leads to a passive escape from the immune response (29). The cytotoxic lymphocytes are suppressed in the tumour microenvironment and follow regulatory mechanisms which inhibit the recognition of tumour antigens by the antigen presenting cells (29). The population of regulatory T cells (Tregs) and the expression of Foxp3 in tumour cells and tumour infiltrating lymphocytes are increased and associated with a suppressed immune system (29).
Immunotherapy and NSCLC
In NSCLC, there have been recent developments on immunotherapies. An example of this are the anti-cytotoxic T-lymphocyte-associated antigen 4 (CTLA-4) inhibitors, like ipilimumab. This consists in a monoclonal antibody (mAb) that blocks CTLA-4 which, in turn, is responsible for balancing the costimulatory signals delivered by CD28 during T-cell activation (17). The CTLA-4 is expressed exclusively on T cells, where it regulates the amplitude of the early stages of the T cells activation. It counteracts the costimulatory receptor CD28, as both have identical ligands: CD80 and CD86 (30). This inhibitor, however, is only approved for the treatment of advanced melanoma and its combination with nivolumab, an anti-programmed cell death-1 (PD-1) inhibitor, is being investigated in NSCLC (31). The anti-PD-1 inhibitors are another option in the treatment of NSCLC. In this case, the mAb blockades the PD-1 checkpoint that targets PD-1, blocking the ligation with its major ligand programmed cell death-ligand 1 (PD-L1) whose overexpression in the tumour is related to better clinical activity of these immunotherapies (17,32,33). As for the PD-1 expression in NSCLC patients, PD-1 negative expression patients, who undergo this treatment, have a similar overall survival when compared with patients treated with chemotherapy (33). In addition, patients with high expression of PD-1 achieve better results with regard to their survival (33). While nivolumab was the first anti-PD-1 checkpoint inhibitor to be approved for metastatic NSCLC patients, pembrolizumab has also been approved for metastatic NSCLC patients whose tumours express PD-L1 (31). The PD-1 is an immune checkpoint normally expressed by the activated T cells, activated Tregs, B cells and even NK cells, but which can also be found on myeloid cells of the stroma surrounding the tumour (17,30,34). It is clear that in comparison with the CTLA-4 this molecule modulates later stages of the immune system response (30). The PD-1 binds to its ligands PD-L1 or programmed cell death-ligand 2 (PD-L2) which are expressed on antigen presenting cells, but can also be found in tumour cells, being an important escape mechanism for them (30). One aspect that must be taken into consideration is the safety use of these therapies, as they can represent general immune system changes in the patients. According to data from many clinical trials, it seems that the CTLA-4 inhibitors are generally safe to use, with manageable side effects related to their mechanism of stimulating the immune system response (35). The therapies involving anti-PD-1 agents seem to be generally similar between each other and the side effects are, in general, manageable (35).
Recently, the immune checkpoint molecule lymphocyte-activation gene 3 (LAG-3) (CD223) and receptor killer-cell immunoglobulin-like receptor (KIR) were reported as possible targets (36). Early data suggest that LAG-3 inhibits T-cell activity, but it modulates T cell activation as well (36). Preclinical data suggests that the anti-tumour activity of PD-1 may work in synergy with LAG-3 (36,37). Lirilumab, a mAb that blocks the interaction between KIR on NK cells and their ligands, can eventually be an option to be used in lung cancer (36). Another option in the treatment of lung cancer is the use of therapeutic vaccines, in which the host’s immune system is forced to recognise tumour antigens, to increase anti-tumour activity by T cells, and to inhibit pathways connected with proliferation, growth, angiogenesis and many other important processes in carcinogenesis (19,38-40). There are two kinds of vaccines: the tumour cell vaccines which are made from autologous or allogeneic tumour cells, and the antigen-based vaccines which are developed to facilitate the exposure of the host’s immune system to tumour-specific antigens (38). Theoretically, the use of vaccines is very effective in minimal residual disease, but there is also a possibility to use them as an adjuvant treatment after resection in an early stage NSCLC, or even as a second line treatment following the chemotherapy and radiation for the advanced stage disease (38).
The incorporation of immunotherapeutic agents, either together or combined with chemotherapy, targeted therapy and radiotherapy, is at the same time a possibility and a challenge (30,41). In an early stage disease, immunotherapy may be combined with a first-line treatments or used as an adjuvant treatment after surgery (42). The combination strategies using chemotherapy, radiation therapy, targeted agents and immunotherapies may become an option against NSCLC, especially when metastasized, but all these possibilities are currently being studied (30). The study of epidermal growth factor receptor (EGFR) or anaplastic lymphoma kinase (ALK) rearrangements may give the patient more options for his treatment (43). Another important aspect is the treatment selection for NSCLC patients, since not all of them will benefit from all therapies (44). Therefore, the finding and study of biomarkers are essential to identify patients who will benefit from these therapies which, in turn, may lead to considerable improvements towards a more personalised and precise medicine (17).
Markers of inflammation and metastasization
MMPs and TIMPs
MMPs are classified as a calcium dependent, Zn2+ containing endopeptidases and can be found in soluble or membrane-bound forms (45). There are 23 human MMPs divided into different groups: collagenases, gelatinases, stromelysins, matrylisins, elastases, membrane-type MMPs and others (45-48). They participate in extracellular matrix (ECM) degradation, but they are also important in tissue homeostasis, host defence, tissue remodelling and repairing, inflammation and many other processes (46,49-51). The proteolytic activity of these molecules is usually very low in healthy tissues, their transcription levels can be upregulated by inflammatory cytokines and growth factors, and their expression can also be regulated by hormones, tumour promoters, cell-to-cell and cell-to-ECM interactions (46). The regulation of their activity is crucial and there are three levels of regulation already reported and studied, namely gene transcription, proenzyme activation and inhibition of enzyme activity (22). The activation requires proteases to remove the propeptide domain, since MMPs are normally secreted as inactive zymogens termed pro-MMPs (22,49,52). Finally, their inhibition can be direct and specific with the TIMPs or non-specific by α1 proteinase inhibitor and α2 macroglobulin (22). Their natural inhibitors, the TIMPs, are specific inhibitors that regulate and control their activities in tissues, and can bind to the MMPs in order to regulate their activity (49,53). There are four TIMPs (TIMP-1-4) identified in vertebrates and their expression is usually upregulated during tissue remodelling (49,53). TIMPs can form complexes with pro-MMPs, so to regulate their action. For example, TIMP-2, TIMP-3 or TIMP-4 can bind with pro-MMP-2 and TIMP-1 or TIMP-3 bind with pro-MMP-9 (53). It is also important to note that the interaction of TIMP-2 with pro-MMP-2 is part of an activation mechanism of pro-MMP-2 into functional MMP-2 and is actually mediated by another MMP, the MMP-14 (53).
In the immune system landscape, MMPs and TIMPs can modify chemotactic agents which are essential for the migration of immune system cells, such as neutrophils (51). For example, MMP-9 processes IL-8 to increase its potency, MMP-2 can inactivate monocyte chemotactic protein-3 (MCP3) which is a cell-attracting chemokine, and MMP-7 mediated cleavage releases C-X-C motif chemokine ligand 3 (CXCL3) which attracts neutrophils to the site of infection (54-56). In neutrophils, MMP-9 is usually stored and ready to release after stimulation with IL-8 or tumour necrosis factor (TNF) (51,57). In mice, TIMP-1-/- neutrophils migrated more rapidly to the site of infection and the immune system response was stronger and amplified; in other study, the neutrophils were suppressed by TIMP-3 (58,59). Another immune system response is given by the macrophages. The M1 or M2 differentiation, described above, is crucial for the type of response given (51). The mRNA levels of MMP-1, MMP-2, MMP-3, MMP-7, MMP-10, MMP-14 and MMP-25 were found to be upregulated and increased in in-vitro M1 macrophages by comparison with the M2 macrophages, suggesting that these MMPs may be important in the M1 differentiation (60).
Elevated expression and activity of MMPs are commonly observed in acute or chronic inflammation, as they can regulate inflammation (61). MMPs can exhibit either pro-inflammatory or anti-inflammatory activities, depending on the characteristics and context. For example, MMP-9 is capable of potentiate the activation of pro-inflammatory cytokines and chemokines, while MMP-2 can process monocyte chemoattractant proteins and, by doing so, it generates chemokine receptor antagonists with anti-inflammatory properties (55,61). MMP-14 was identified as an important MMP for the regulation of pro-inflammatory cytokines, such as the IL-12 and IL-6 as well as the anti-inflammatory IL-10; it can also cleave and inactivate chemokines which are involved in inflammation (51). In the inflammation of the airways, the ECM degradation generates peptides, which exhibit chemotactic activity through the activation of CXC chemokine receptor on neutrophilic granulocytes, and the MMP-12 cleavage of neutrophil elastase can generate neutrophil attracting peptides (46,62). MMPs can also compromise the T cell activity, since several MMPs can cleave transforming growth factor β (TGF-β) to its active form which inhibits T cell response (63). MMP-9 produced by tumour cells can cleave IL-2Rα on the surface of activated T cells and suppress their activity and their proliferative capacity (63).
In the cancer landscape, MMPs are believed to facilitate metastasis by breaking down physical barriers provided by ECM or by the basement membrane, but their action in carcinogenesis is important in many other processes, such as tumour growth, apoptosis and angiogenesis (22,64). MMPs are able to regulate tumour growth by many mechanisms: they can release cell membrane bound precursors of growth factors, they are able to modulate the bioavailability of growth factors sequestered by ECM proteins, and they can indirectly modulate proliferative signals (22). Many MMPs are capable of activating cell surface bound TNF-α which promotes cell survival via NF-κB pathway (46). MMP-8, MMP-9 or MMP-12 can modulate the activity of CXCL11, which is a Th1 attracting chemokine, whose receptor (CXCR7) is expressed in many types of tumour cells and activates signalling pathways that promote cell survival and growth (65). However, MMPs are also able to inhibit growth by activating TGF-β, generating proapoptotic molecules, such as the Fas ligand (22,66). They may, critically, disrupt the balance between growth and antigrowth signals in tumour microenvironment, due to their potential to influence bioavailability and functionality of multiple important factors regulating growth (52). In the apoptotic process, MMPs also have dual roles. Antiapoptotic actions are mediated by the cleaving of the Fas ligand, proteolytic shedding of tumour associated MHC complex class I related protein and activation of serine, threonine kinases or protein kinase B (22). The MMPs’ apoptotic actions involve the changing of ECM composition by their ability to cleave adhesion molecules and, eventually, lead the cell to apoptosis (22,67). MMPs interfere with the induction of apoptosis in malignant cells and can even lower the impact of chemotherapy on the tumour (52). MMPs also play a dual role in Angiogenesis, as they can either promote or inhibit it. They are able to promote endothelial cell migration and trigger the angiogenic switch (61). Pro-angiogenic MMPs are normally produced by stromal cells or inflammatory leukocytes (64). They can promote angiogenesis by degradation of basement membrane and other ECM components, by releasing ECM bound pro-angiogenic factors [such as the vascular endothelial growth factor (VEGF)], and by triggering integrin intracellular signalling (22,68). They are capable of inhibiting angiogenesis by cleaving plasminogen, which releases angiostatin; by cleaving collagen, which produces endostatin; and by shedding cell surface bound plasminogen activator receptors, which are required for endothelial cell invasion (22,52,67-70).
The proteolytic activity of MMPs is essential to allow the malignant cell to break physical barriers during expansion and intravasation, extravasation and invasion at distant sites (67). Hence, MMPs are strongly associated with metastasization (67). During invasion, MMPs can be found in structures called invadopodia, which represent the site where ECM degradation will take place (22,67). MMP-14 and MMP-1, -2 or -9 are examples of MMPs that degrade a variety of ECM molecules and facilitate invasion (22,67). Many proteolytic enzymes are fundamental for tumour cells to access blood vessels and lymphatic system; MMP-2 and MMP-9 can both degrade components of basement membrane, enhancing the invasion process (22). It is also described that MMPs are important factors for circulatory tumour cells to extravasate and establish colonies (22). The cell movement is also associated with MMPs, as they can regulate cell-cell and cell-ECM interactions during migration (67). The production of peptides, such as collagen and laminin, can also promote cell migration, due to the degradation of ECM components or integrins that act as substrates for MMPs (67,71-73). MMP-2 and MMP-14 degrade laminin 5 and trigger motility of the cell (22). Epithelial-mesenchymal transition (EMT) has also been associated with high expression levels of MMPs, such as MMP-2,-7,-9 among others (66,67). Their activity is important in this crucial process for the metastasization to occur (22). For example, MMP-7 is capable of cleaving E-cadherin disrupting cell adhesion and leading to the EMT and migration (22,67,74). It is also described that the EMT can be induced by TGF-β and that MMP-28 can cause its proteolytic activation and lead to EMT (22,75). E-cadherin can be cleaved by MMP-3 and MMP-7 and it triggers EMT (22). In general, studies show that overexpression of some MMPs conferred tumour cells with more tumorigenicity and invasiveness (64).
Usually, high MMPs activity is correlated with tumour progression, but the balance between them and their inhibitors is crucial. Deregulation of TIMPs may result in an increase of MMPs activities and, thereby, in a more invasive potential for tumour cells. Upregulation of TIMPs can actually inhibit tumour invasion and metastasis (61). However, their action is not only associated with tumour suppressing activities, although it is reported that TIMPs commonly act as tumour suppressive agents and are normally dysregulated in cancer landscape, which can justify the conflicting data reports (61).
In NSCLC, many studies have been developed with promising results, demonstrating that these biomolecules can be useful and potentially be considered biomarkers, as they can eventually predict clinical outcomes, such as prognosis or drug response, which demonstrates their importance in both carcinogenesis, as described above, and in the future of personalised medicine. High expression in tumour and peritumoural cells of MMP-2 was associated with increased risk of tumour recurrence and with poor prognosis (76). Its overexpression in patients at an early stage was also observed and was related to poor prognosis, as well as to invasion and metastasis (77,78). Its serum levels are usually higher in patients with metastases than in patients with localised disease being, thereby, associated with poor prognosis, and can eventually be used to monitor the progression of the disease (79). High MMP-7 expression in NSCLC is common and was already associated with poor response to platinum-derived chemotherapy, showing potential to be used in these patients, as a predictive drug response marker for the resistance to chemotherapy (52,80,81). The expression and activity of MMP-9 is usually upregulated and it seems to be related to the clinical stage of the patient, tumour growth, disease progression and aggressive tumour behaviour, leaving open the possibility for it to be used as a prognosis marker and a therapeutic target (81-85). TIMPs are also important due to their relationship with MMPs, and their importance in NSCLC has also been studied over the years. TIMP-1 mRNA expression levels in tumour tissue are usually higher by comparison with normal lung tissue (86). Its expression has also been associated with decreased survival, low recurrence-free survival and poor prognosis, which can possibly happen due to the context and other TIMP-1 activities besides MMP inhibition (87-90). The role of this TIMP in NSCLC is still to be fully discovered and additional studies will provide a better insight into its functions in cancer development (87-90). As TIMP-2 expression indicates a favourable prognosis in patients with NSCLC, it may be a protective factor that could help predict the outcome of chemotherapy or targeted inhibitory antibody therapies (91).
MMPs were already looked upon as potential therapeutic targets, but their dual role is one of the possible reasons why the outcome of some clinical trials with MMP inhibitors was not desirable (46). In NSCLC, a phase III trial with marimastat, a synthetic MMP inhibitor based on hydroxamate which acts as a zinc-binding group, observed no significant difference, in progression-free survival or overall survival, between the group with the inhibitor and the group with the placebo (61). However, prinomastat, an alternative zinc-binding non-peptide MMP inhibitor, demonstrated antitumor activities in various preclinical studies, including lung cancer models (61). In particular, in NSCLC the anti-tumour efficacy in chemoresistant human NSCLC was observed with prinomastat as a single agent or when combined with chemotherapy (61). On the contrary, a phase III trial with a combination of taxol and carboplatin for advanced NSCLC patients was not effective (61). Nevertheless, the possibility of being a good option in early stages remains unknown (61). A different therapeutic approach is the use of nanoparticles micelles containing paclitaxel conjugated to a hydrophilic moiety via an MMP-2 cleavable peptide (92). These nanoparticles were effective in NSCLC xenografts by comparison with paclitaxel or micelles alone (92). This efficacy may be justified due to the accumulation of micelles at the tumour site, by virtue of the enhanced permeability and retention (EPR) effect and of the peptide cleavage by MMP-2 leading to active cell penetrating paclitaxel (92). In lung cancer carcinoma cells, resistance to different platinum compound or cisplatin after exposure to MMP-7 was related to increased levels of anti-apoptotic protein BCL2, which means that this MMP inhibition may be used as adjunct to chemotherapy for those who show some resistance (92).
In summary, there are many processes involved in carcinogenesis, where MMPs play important roles and their activity can influence them, as shown in Table 1 (61,67,93).
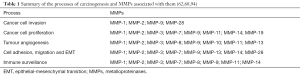
Full table
ILs
It is known that the mediators of inflammation and immune system response are, in general, very important in the tumour microenvironment. The inflammation registered has many tumour promoting effects, as it promotes angiogenesis, tumour growth. progression and metastasis, thereby altering the immune response (25,94). One of those mediators are cytokines, which have dual roles: they have influence in changing the balance from an active and tumour eliminating immunosurveillance status to a tumour promoting microenvironment, and they can also have tumour suppressing effects (95). In this context, attention will be paid to one major class of cytokines, the ILs, crucial components of both the adaptive and innate immune system response (96,97). They are considered to be cellular messenger molecules, because they allow cells of the immune system to communicate between each other and to create coordinated, specific responses to an antigen (23,98). They act in a paracrine or autocrine way and the response of a single cell to them depends on the ligands involved, the receptors expressed on the cell surface and the many cascades that can be involved (99). Almost all ILs are also capable of modulating growth differentiation and activation during immune system response and they can have both pro-inflammatory or anti-inflammatory functions (23,99-101).
During tumorigenesis, ILs can stimulate immune system effector cells and stromal cells at the tumour site, in order to enhance the tumour cell recognition by the cytotoxic effector cells (23). They are also involved, directly or indirectly, in many mechanisms of the tumour development, such as immunoediting, immunosurveillance, inflammation and EMT mechanisms (23). The role of ILs in immunosurveillance and immunoediting is crucial in cancer landscape and may lead to new opportunities and possibilities of novel therapeutic approaches in the future, but due to their activity and function in the immune system and many molecular pathways, their use for cancer treatment may also have some limitations, such as severe immunological adverse conditions (23,102).
In the NSCLC landscape, the roles of many ILs and their importance in this cancer have been studied over the years, as shown by the examples listed in Table 2 (103-118).
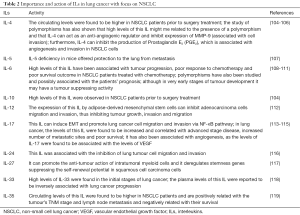
Full table
Anti-inflammatory ILs can also inhibit angiogenesis, growth and invasion, as is the case of the IL-4, usually produced by T cells, mast cells, basophils and is capable of inducing antibody production by B cells, (104). High levels in NSCLC support the hypothesis that Th2 cytokines associated with the inhibition of lymphocyte activation are usually higher in these patients and Th17 related to cytokines are also increased by comparison with controls (103,119). However, this interleukin and its characteristics were also associated with potential inhibitory features that may act against the tumour (104,105). In fact, some ILs may exhibit anti-tumour roles. IL-24 can disrupt stromal cell-derived factor 1 (SDF-1)/CXCR4 signalling pathway and, by doing so, it can inhibit lung tumour cell migration and invasion; and when combined with CXCR4 inhibitors, it exhibits anti-metastatic activities (115). IL-27 has also been associated with anti-tumour effects, such as anti-proliferation and anti-angiogenesis, as well as with immune system stimulation (116). The study of IL-33, associated with the induction of Th2 cytokines, can inhibit infection and inflammation, this supports the hypothesis of a tumour suppressor microenvironment present in cancer patients, at least at early stages of the development, with plasma levels of this interleukin being inversely associated with lung cancer progression (117). It is also known that Treg cells Foxp3+ are capable of producing IL-35 and that this interleukin is required for T-reg mediated immunosuppression, as well as it is important in T cell proliferation suppression and it can induce naïve T cells to convert into Treg cells (118). In NSCLC, circulating levels of this interleukin are increased and associated with poor overall survival (118).
On the other hand, pro-inflammatory ILs, such as the IL-6 which can be produced by many immune system cells in acute or chronic inflammation, can act as a growth and an antiapoptotic factor (109,120). High levels of this interleukin have been associated with a poor prognosis, therapeutic resistance and metastatic disease (120-122). However, it may also have an anti-tumour activity, at least at very early stages of tumour development induced by K-Ras (110). Another example is given by IL-17, a vital interleukin in chronic inflammation, but also very important in the pathophysiology of cancer itself (112,114). It is capable of inducing EMT and of promoting lung cancer cell migration and invasion via NF-κB pathway (112). It has been also associated with angiogenesis, with a more advanced stage of the disease and with worse overall survival (113,114).
ILs associated with allergic and infectious diseases, such as the IL-5, have also been studied in mice lung metastasis models (106). It was suggested that IL-5 may facilitate metastasis and colonisation through the recruitment of eosinophils, which produce C-C motif chemokine 22 (CCL22), leading to the recruitment of Treg cells to its location and, therefore, to a regulation of the immune system in the tumour microenvironment (106).
Although the relationship between ILs and MMPs has also been studied, more insight is needed so to clarify and support these and other relations, specifically in the NSCLC landscape, as most of the studies rely on several types of in vitro and in vivo studies. Clinical studies focusing on the levels of ILs and MMPs and their relationship will give more insight in this matter and on the clinical importance of these findings. IL-1 is believed to play an important role in tumour promotion and metastasis through the regulation and promotion of expression of MMPs (23,102). For example, Il-1β may upregulate the expression of MMP-13 (23). In bladder cancer cell lines IL-5 was associated with invasion and MMP-9 expression (102). The stimulation of IL-6 can also induce an elevated expression of MMP-2 and MMP-9 in nasopharyngeal carcinoma cell lines (123). In lung cancer cell lines, IL-6 was reported to regulate both the expression of mRNA and the protein levels of MMP-10 (124). To be noted that high levels of IL-6 were also related to high expression of MMP-3, MMP-13, thereby enhancing the migration abilities of these cells (125). IL-8 and IL-17 enhance the activity of MMP-2 and MMP-9 which, in turn, increases the metastatic activity and as for IL-17, this IL was already associated with the expression and activity of MMPs; it induces the expression of MMP-9 in vivo and in vitro and its inhibition in tumour sites may suppress MMP-9 (23,114,126,127) and IL-17A, in a gastric cancer cell line, promoted an elevated expression of MMP-2 and MMP-9 as well as a diminishing expression of TIMP-1 and TIMP-2 levels, therefore enhancing invasion (128). High levels of IL-23 have also been associated with an upregulation of MMP-9, related to an increase of angiogenesis, which appears to be important in tumour-promoting pro-inflammatory processes (97). High expression of IL-32 is also associated with cell migration and invasion in vitro, as it can upregulate the expression of MMP-2 and MMP-9 (129). It is also interesting to note that MMPs can also potentiate the activation of cytokines, due to their activity. For example, MMP-9 can potentiate the activation of pro-inflammatory cytokines, such as TNF, IL-1β, IL-6, IL-8, and chemokines (61).
As aforementioned, ILs have important roles in carcinogenesis. Their influence in processes, such as inflammation, angiogenesis or metastasis, is crucial and very important in order to regulate the tumour microenvironment and the immune system response. They can exhibit either pro or anti-tumour effects, and some of them may have potential to be considered as a prognosis marker or even a therapeutic agent with therapeutic potential. Their immunomodulatory functions are essential in immunosurveillance and their roles can difficult their targeting, as it may change the overall function and homeostasis of the patient’s immune system.
Conclusions
The relationship between the immune system and NSCLC has been studied over the years. The tumour development seems to be associated with a local inflammation which can later on become chronic. Although at the beginning was believed that the immune system acted against the tumour, from a certain moment onwards that reaction was altered, modulated, and eventually it was concluded that the immune microenvironment helps the tumour to expand and develop. The current knowledge on the immune checkpoints have already enabled us to have immunotherapies showing promising results in this cancer. The finding of the loss of standard biomarkers allowed the scientific community not only to develop new targeted therapies, but also to have a better insight on the NSCLC patients and their prognosis or treatment response. However, this cancer continues to be the deadliest. As such, the finding of new biomarkers, which can help understand which patients have the better treatment response or their prognosis, is particularly important. Here, the markers of inflammation and metastasization are potential candidates to be looked upon as important biomarkers in this cancer. Metalloproteinases and their inhibitors as well as many ILs have already been studied and many of them were associated with poor prognosis or differences in the treatment response. Despite the potential, there are still many obstacles and limitations in incorporating these biomarkers into clinical practice since, as seen above, MMPs, TIMPs and ILs play dual roles in carcinogenesis, having pro or anti-tumoural effects. The future perspective in this area certainly involves a better knowledge on the MMPs, TIMPs and ILs mechanisms and moments of action. Therefore, it is important to understand what triggers the tumour cells to evade the immune system response and how are they able to use its intermediates and their surrounding environment to actually develop and expand. The complex networks in which these markers are involved are also a problem, in order to fully understand their action and what triggers their reaction. Nonetheless, what truly stands for the potential of these markers is the possibility to use them as markers of prognosis or of treatment response. Eventually, the development of new studies, which gather a considerable number of NSCLC patients, focused on understanding the importance of MMPS, TIMPs and ILs in the prognosis and treatment response of NSCLC patients, will improve our knowledge on the subject and will clearly show if they can be used in clinical practice. Although the potential of some MMPs, TIMPs and ILs has already been exploited and studied, with promising results seeming to offer a possibility of their use in the future, new studies are needed to clarify and actually verify the results that have been reported.
Acknowledgments
Funding: None.
Footnote
Conflicts of Interest: All authors have completed the ICMJE uniform disclosure form (available at http://dx.doi.org/10.21037/tcr.2018.05.16). The authors have no conflicts of interest to declare.
Ethical Statement: The authors are accountable for all aspects of the work in ensuring that questions related to the accuracy or integrity of any part of the work are appropriately investigated and resolved.
Open Access Statement: This is an Open Access article distributed in accordance with the Creative Commons Attribution-NonCommercial-NoDerivs 4.0 International License (CC BY-NC-ND 4.0), which permits the non-commercial replication and distribution of the article with the strict proviso that no changes or edits are made and the original work is properly cited (including links to both the formal publication through the relevant DOI and the license). See: https://creativecommons.org/licenses/by-nc-nd/4.0/.
References
- Hanahan D, Weinberg RA. Hallmarks of cancer: The next generation. Cell 2011;144:646-74. [Crossref] [PubMed]
- Bremnes RM, Al-Shibli K, Donnem T, et al. The role of tumor-infiltrating immune cells and chronic inflammation at the tumor site on cancer development, progression, and prognosis: emphasis on non-small cell lung cancer. J Thorac Oncol 2011;6:824-33. [Crossref] [PubMed]
- Dunn GP, Bruce AT, Ikeda H, et al. Cancer immunoediting: from immunosurveillance to tumor escape. Nat Immunol 2002;3:991-8. [Crossref] [PubMed]
- Carosella ED, Favier B, Rouas-freiss N, et al. Beyond the increasing complexity of the immunomodulatory HLA-G molecule. Blood 2008;111:4862-70. [Crossref] [PubMed]
- Remark R, Becker C, Gomez JE, et al. The Non-Small Cell Lung Cancer Immune Contexture. A Major Determinant of Tumor Characteristics and Patient Outcome. Am J Respir Crit Care Med 2015;191:377-90. [Crossref] [PubMed]
- Herbst RS, Heymach JV, Lippman SM. Lung cancer. N Engl J Med 2008;359:1367-80. [Crossref] [PubMed]
- Hwang SJ, Cheng LS, Lozano G, et al. Lung cancer risk in germline p53 mutation carriers: association between an inherited cancer predisposition, cigarette smoking, and cancer risk. Hum Genet 2003;113:238-43. [Crossref] [PubMed]
- Sanders BM, Jay M, Draper GJ, et al. Non-ocular cancer in relatives of retinoblastoma patients. Br J Cancer 1989;60:358-65. [Crossref] [PubMed]
- Bell DW, Gore I, Okimoto RA, et al. Inherited susceptibility to lung cancer may be associated with the T790M drug resistance mutation in EGFR. Nat Genet 2005;37:1315-6. [Crossref] [PubMed]
- Spitz MR, Wei Q, Dong Q, et al. Genetic susceptibility to lung cancer: the role of DNA damage and repair. Cancer Epidemiol Biomarkers Prev 2003;12:689-98. [PubMed]
- Davidson MR, Gazdar AF, Clarke BE. The pivotal role of pathology in the management of lung cancer. J Thorac Dis 2013;5:S463-78. [PubMed]
- Langer CJ, Besse B, Gualberto A, et al. The Evolving Role of Histology in the Management of Advanced Non-Small-Cell Lung Cancer. J Clin Oncol 2010;28:5311-20. [Crossref] [PubMed]
- Aisner DL, Marshall CB. Molecular pathology of non-small cell lung cancer: A practical guide. Am J Clin Pathol 2012;138:332-46. [Crossref] [PubMed]
- Chen Z, Fillmore CM, Hammerman PS, et al. Non-small-cell lung cancers: a heterogeneous set of diseases. Nat Rev Cancer 2014;14:535-46. [Crossref] [PubMed]
- Davies H, Bignell GR, Cox C, et al. Mutations of the BRAF gene in human cancer. Nature 2002;417:949-54. [Crossref] [PubMed]
- Yu Y, He J. Molecular classification of non-small-cell lung cancer: diagnosis, individualized treatment, and prognosis. Front Med 2013;7:157-71. [Crossref] [PubMed]
- Brahmer JR, Pardoll DM. Immune checkpoint inhibitors: making immunotherapy a reality for the treatment of lung cancer. Cancer Immunol Res 2013;1:85-91. [Crossref] [PubMed]
- Hayes DF, Schott AF. Personalized Medicine: Genomics Trials in Oncology. Trans Am Clin Climatol Assoc 2015;126:133-43. [PubMed]
- Moreira AL, Eng J. Personalized therapy for lung cancer. Chest 2014;146:1649-57. [Crossref] [PubMed]
- Spaans JN, Goss GD. Drug resistance to molecular targeted therapy and its consequences for treatment decisions in non-small-cell lung cancer. Front Oncol 2014;4:190. [Crossref] [PubMed]
- Fang B, Mehran RJ, Heymach JV, et al. Predictive biomarkers in precision medicine and drug development against lung cancer. Chin J Cancer 2015;34:295-309. [Crossref] [PubMed]
- Yadav L, Puri N, Rastogi V, et al. Matrix metalloproteinases and cancer - Roles in threat and therapy. Asian Pac J Cancer Prev 2014;15:1085-91. [Crossref] [PubMed]
- Anestakis D, Petanidis S, Kalyvas S, et al. Mechanisms and applications of Interleukins in cancer immunotherapy. Int J Mol Sci 2015;16:1691-710. [Crossref] [PubMed]
- Allavena P, Sica A, Solinas G, et al. The inflammatory micro-environment in tumor progression: The role of tumor-associated macrophages. Crit Rev Oncol Hematol 2008;66:1-9. [Crossref] [PubMed]
- Mantovani A, Allavena P, Sica A, et al. Cancer-related inflammation. Nature 2008;454:436-44. [Crossref] [PubMed]
- Thomas A, Giaccone G. Why has active immunotherapy not worked in lung cancer? Ann Oncol 2015;26:2213-20. [Crossref] [PubMed]
- Dunn GP, Koebel CM, Schreiber RD. Interferons, immunity and cancer immunoediting. Nat Rev Immunol 2006;6:836-48. [Crossref] [PubMed]
- Chan CW, Tsui SK, Law BM, et al. The Utilization of the Immune System in Lung Cancer Treatment: Beyond Chemotherapy. Int J Mol Sci 2016;17:286. [Crossref] [PubMed]
- Domagala-Kulawik J. The role of the immune system in non-small cell lung carcinoma and potential for therapeutic intervention. Transl Lung Cancer Res 2015;4:177-90. [PubMed]
- Champiat S, Ileana E, Giaccone G, et al. Incorporating immune-checkpoint inhibitors into systemic therapy of NSCLC. J Thorac Oncol 2014;9:144-53. [Crossref] [PubMed]
- Le AD, Alzghari SK, Jean GW, et al. Update on targeted therapies for advanced non-small cell lung cancer: Nivolumab in context. Ther Clin Risk Manag 2017;13:223-36. [Crossref] [PubMed]
- Passiglia F, Bronte G, Bazan V, et al. PD-L1 expression as predictive biomarker in patients with NSCLC: a pooled analysis. Oncotarget 2016;7:19738-47. [Crossref] [PubMed]
- Rolfo C, Caglevic C, Santarpia M, et al. Immunotherapy in NSCLC: A Promising and Revolutionary Weapon. In: Naing A, Hajjar J. Editors. Immunotherapy. Cham: Springer International Publishing, 2017;97-125.
- Pardoll DM. The blockade of immune checkpoints in cancer immunotherapy. Nat Rev Cancer 2012;12:252-64. [Crossref] [PubMed]
- Shih K, Arkenau H, Infante JR. Clinical Impact of Checkpoint Inhibitors as Novel Cancer Therapies. Drugs 2014;74:1993-2013. [Crossref] [PubMed]
- Heigener D, Reck M. Exploring the potential of immuno-oncology-based treatment for patients with non-small cell lung cancer. Expert Rev Anticancer Ther 2015;15:69-83. [Crossref] [PubMed]
- Sierro S, Romero P, Speiser DE. The CD4-like molecule LAG-3, biology and therapeutic applications. Expert Opin Ther Targets 2011;15:91-101. [Crossref] [PubMed]
- Rangachari D, Brahmer JR. Targeting the immune system in the treatment of non-small-cell lung cancer. Curr Treat Options Oncol 2013;14:580-94. [Crossref] [PubMed]
- Jang SJ, Soria JC, Wang L, et al. Activation of melanoma antigen tumor antigens occurs early in lung carcinogenesis. Cancer Res 2001;61:7959-63. [PubMed]
- Gonzalez G, Crombet T, Torres F, et al. Epidermal growth factor-based cancer vaccine for non-small-cell lung cancer therapy. Ann Oncol 2003;14:461-6. [Crossref] [PubMed]
- Sundar R, Soong R, Cho BC, et al. Immunotherapy in the treatment of non-small cell lung cancer. Lung Cancer 2014;85:101-9. [Crossref] [PubMed]
- Suzuki H, Owada Y, Watanabe Y, et al. Recent advances in immunotherapy for non-small-cell lung cancer. Hum Vaccin Immunother 2014;10:352-7. [Crossref] [PubMed]
- Kris MG, Johnson BE, Berry LD, et al. Using multiplexed assays of oncogenic drivers in lung cancers to select targeted drugs. JAMA 2014;311:1998-2006. [Crossref] [PubMed]
- Declerck S, Vansteenkiste J. Immunotherapy for lung cancer: ongoing clinical trials. Future Oncol 2014;10:91-105. [Crossref] [PubMed]
- Pulkoski-Gross AE. Historical Perspective of Matrix Metalloproteases. Front Biosci (Schol Ed) 2015;7:125-49. [Crossref] [PubMed]
- Nissinen L, Kähäri VM. Matrix metalloproteinases in inflammation. Biochim Biophys Acta 2014;1840:2571-80. [Crossref] [PubMed]
- Nagase H, Visse R, Murphy G. Structure and function of matrix metalloproteinases and TIMPs. Cardiovasc Res 2006;69:562-73. [Crossref] [PubMed]
- Hua Y, Nair S. Proteases in cardiometabolic diseases: Pathophysiology, molecular mechanisms and clinical applications. Biochim Biophys Acta 2015;1852:195-208. [Crossref] [PubMed]
- Visse R, Nagase H. Matrix metalloproteinases and tissue inhibitors of metalloproteinases: Structure, function, and biochemistry. Circ Res 2003;92:827-39. [Crossref] [PubMed]
- Sternlicht M, Werb Z. How Matrix metalloproteinases regulate cell behavior. Annu Rev Cell Dev Biol 2001;17:463-516. [Crossref] [PubMed]
- Khokha R, Murthy A, Weiss A. Metalloproteinases and their natural inhibitors in inflammation and immunity. Nat Rev Immunol 2013;13:649-65. [Crossref] [PubMed]
- Kessenbrock K, Plaks V, Werb Z. Matrix Metalloproteinases: Regulators of the Tumor Microenvironment. Cell 2010;141:52-67. [Crossref] [PubMed]
- Brew K, Nagase H. The tissue inhibitors of metalloproteinases (TIMPs): An ancient family with structural and functional diversity. Biochim Biophys Acta 2010;1803:55-71. [Crossref] [PubMed]
- Van den Steen PE, Proost P, Wuyts A, et al. Neutrophil gelatinase B potentiates interleukin-8 tenfold by aminoterminal processing, whereas it degrades CTAP-III, PF-4, and GRO-alpha and leaves RANTES and MCP-2 intact. Blood 2000;96:2673-81. [PubMed]
- McQuibban CA, Gong JH, Tam EM, et al. Inflammation Dampened by Gelatinase A Cleavage of Monocyte Chemoattractant Protein-3. Science 2000;289:1202-6. [Crossref] [PubMed]
- Li Q, Park PW, Wilson CL, et al. Matrilysin shedding of syndecan-1 regulates chemokine mobilization and transepithelial efflux of neutrophils in acute lung injury. Cell 2002;111:635-46. [Crossref] [PubMed]
- Chakrabarti S, Patel KD. Regulation of matrix metalloproteinase-9 release from IL-8-stimulated human neutrophils. J Leukoc Biol 2005;78:279-88. [Crossref] [PubMed]
- Kim KH, Burkhart K, Chen P, et al. Tissue inhibitor of metalloproteinase-1 deficiency amplifies acute lung injury in bleomycin-exposed mice. Am J Respir Cell Mol Biol 2005;33:271-9. [Crossref] [PubMed]
- Gill SE, Huizar I, Bench EM, et al. Tissue inhibitor of metalloproteinases 3 regulates resolution of inflammation following acute lung injury. Am J Pathol 2010;176:64-73. [Crossref] [PubMed]
- Huang WC, Sala-Newby GB, Susana A, et al. Classical macrophage activation up-regulates several matrix metalloproteinases through mitogen activated protein kinases and nuclear factor-kB. PLoS One 2012;7:1-14.
- Hua H, Li M, Luo T, et al. Matrix metalloproteinases in tumorigenesis: An evolving paradigm. Cell Mol Life Sci 2011;68:3853-68. [Crossref] [PubMed]
- Weathington NM, van Houwelingen AH, Noerager BD, et al. A novel peptide CXCR ligand derived from extracellular matrix degradation during airway inflammation. Nat Med 2006;12:317-23. [Crossref] [PubMed]
- Sheu BC, Hsu SM, Ho HN, et al. A novel role of metalloproteinase in cancer-mediated immunosuppression. Cancer Res 2001;61:237-42. [PubMed]
- Deryugina EI, Quigley JP. Matrix metalloproteinases and tumor metastasis. Cancer Metastasis Rev 2006;25:9-34. [Crossref] [PubMed]
- Burns JM, Summers BC, Wang Y, et al. A novel chemokine receptor for SDF-1 and I-TAC involved in cell survival, cell adhesion, and tumor development. J Exp Med 2006;203:2201-13. [Crossref] [PubMed]
- Egeblad M, Werb Z. New functions for the matrix metalloproteinases in cancer progression. Nat Rev Cancer 2002;2:161-74. [Crossref] [PubMed]
- Gialeli C, Theocharis AD, Karamanos NK. Roles of matrix metalloproteinases in cancer progression and their pharmacological targeting. FEBS J 2011;278:16-27. [Crossref] [PubMed]
- Rundhaug JE. Matrix metalloproteinases and angiogenesis. J Cell Mol Med 2005;9:267-85. [Crossref] [PubMed]
- Ferreras M, Felbor U, Lenhard T, et al. Generation and degradation of human endostatin proteins by various proteinases. FEBS Lett 2000;486:247-51. [Crossref] [PubMed]
- Koolwijk P, Sidenius N, Peters E, et al. Proteolysis of the urokinase-type plasminogen activator receptor by metalloproteinase-12: Implication for angiogenesis in fibrin matrices. Blood 2001;97:3123-31. [Crossref] [PubMed]
- Koshikawa N, Giannelli G, Cirulli V, et al. Role of cell surface metalloprotease MT1-MMP in epithelial cell migration over laminin-5. J Cell Biol 2000;148:615-24. [Crossref] [PubMed]
- Xu J, Rodriguez D, Petitclerc E, et al. Proteolytic exposure of a cryptic site within collagen type IV is required for angiogenesis and tumor growth in vivo. J Cell Biol 2001;154:1069-79. [Crossref] [PubMed]
- Baciu PC, Suleiman EA, Deryugina EI, et al. Membrane type-1 matrix metalloproteinase (MT1-MMP) processing of pro-alphav integrin regulates cross-talk between alphavbeta3 and alpha2beta1 integrins in breast carcinoma cells. Exp Cell Res 2003;291:167-75. [Crossref] [PubMed]
- Noë V, Fingleton B, Jacobs K, et al. Release of an invasion promoter E-cadherin fragment by matrilysin and stromelysin-1. J Cell Sci 2001;114:111-8. [PubMed]
- Illman SA, Lehti K, Keski-Oja J, et al. Epilysin (MMP-28) induces TGF-beta mediated epithelial to mesenchymal transition in lung carcinoma cells. J Cell Sci 2006;119:3856-65. [Crossref] [PubMed]
- Leinonen T, Pirinen R, Böhm J, et al. Increased expression of matrix metalloproteinase-2 (MMP-2) predicts tumour recurrence and unfavourable outcome in non-small cell lung cancer. Histol Histopathol 2008;23:693-700. [PubMed]
- Passlick B, Sienel W, Seen-Hibler R, et al. Overexpression of matrix metalloproteinase 2 predicts unfavorable outcome in early-stage non-small cell lung cancer. Clin Cancer Res 2000;6:3944-8. [PubMed]
- Li GH, Cui YS, Wu QY, et al. Clinicopathologic significance of beta-catenin and matrix metalloproteinase-2 expression in non-small cell lung cancer. Med Oncol 2013;30:437. [Crossref] [PubMed]
- Kanoh Y, Abe T, Masuda N, et al. Progression of non-small cell lung cancer: Diagnostic and prognostic utility of matrix metalloproteinase-2, C-reactive protein and serum amyloid A. Oncol Rep 2013;29:469-73. [Crossref] [PubMed]
- Liu H, Zhang T, Li X, et al. Predictive value of MMP-7 expression for response to chemotherapy and survival in patients with non-small cell lung cancer. Cancer Sci 2008;99:2185-92. [Crossref] [PubMed]
- Leinonen T, Pirinen R, Böhm J, et al. Expression of matrix metalloproteinases 7 and 9 in non-small cell lung cancer: Relation to clinicopathological factors, β-catenin and prognosis. Lung Cancer 2006;51:313-21. [Crossref] [PubMed]
- El-badrawy MK, Yousef AM, Shaalan D, et al. Matrix Metalloproteinase-9 Expression in Lung Cancer Patients and Its Relation to Serum MMP-9 Activity, Pathologic Type, and Prognosis. J Bronchology Interv Pulmonol 2014;21:327-34. [Crossref] [PubMed]
- Hrabec E, Strek M, Nowak D, et al. Elevated level of circulating matrix metalloproteinase-9 in patients with lung cancer. Respir Med 2001;95:1-4. [Crossref] [PubMed]
- Peng WJ, Zhang JQ, Wang BX, et al. Prognostic value of matrix metalloproteinase 9 expression in patients with non-small cell lung cancer. Clin Chim Acta 2012;413:1121-6. [Crossref] [PubMed]
- Wang JL, Wu DW, Cheng ZZ, et al. Expression of high mobility group box - B1 (HMGB-1) and matrix metalloproteinase-9 (MMP-9) in non-small cell lung cancer (NSCLC). Asian Pac J Cancer Prev 2014;15:4865-9. [Crossref] [PubMed]
- Safranek J, Pesta M, Holubec L, et al. Expression of MMP-7, MMP-9, TIMP-1 and TIMP-2 mRNA in lung tissue of patients with non-small cell lung cancer (NSCLC) and benign pulmonary disease. Anticancer Res 2009;29:2513-7. [PubMed]
- Aljada IS, Ramnath N, Donohue K, et al. Upregulation of the tissue inhibitor of metalloproteinase-1 protein is associated with progression of human non-small-cell lung cancer. J Clin Oncol 2004;22:3218-29. [Crossref] [PubMed]
- An HJ, Lee YJ, Hong SA, et al. The prognostic role of tissue and serum MMP-1 and TIMP-1 expression in patients with non-small cell lung cancer. Pathol Res Pract 2016;212:357-64. [Crossref] [PubMed]
- Pesta M, Kulda V, Kucera R, et al. Prognostic Significance of TIMP-1 in Non-small Cell Lung Cancer. Anticancer Res 2011;31:4031-8. [PubMed]
- Suemitsu R, Yoshino I, Tomiyasu M, et al. Serum tissue inhibitors of metalloproteinase-1 and -2 in patients with non-small cell lung cancer. Surg Today 2004;34:896-901. [Crossref] [PubMed]
- Zhu L, Yu H, Liu S, et al. Prognostic Value of Tissue Inhibitor of Metalloproteinase-2 Expression in Patients with Non–Small Cell Lung Cancer: A Systematic Review and Meta-Analysis. PloS One 2015;10:e0124230 [Crossref] [PubMed]
- Shay G, Lynch CC, Fingleton B. Moving targets: Emerging roles for MMPs in Cancer Progression and Metastasis. Matrix Biol 2015;44-46:200-6. [Crossref] [PubMed]
- Gomes M, Teixeira AL, Coelho A, et al. The Role of Inflammation in Lung Cancer. Adv Exp Med Biol 2014;816:1-23. [Crossref] [PubMed]
- Shalapour S, Karin M. Immunity, inflammation, and cancer: An eternal fight between good and evil. J Clin Invest 2015;125:3347-55. [Crossref] [PubMed]
- Burkholder B, Huang RY, Burgess R, et al. Tumor-induced perturbations of cytokines and immune cell networks. Biochim Biophys Acta 2014;1845:182-201. [PubMed]
- Kaiser P, Rothwell L, Avery S, et al. Evolution of the interleukins. Dev Comp Immunol 2004;28:375-94. [Crossref] [PubMed]
- Meager A, Wadhwa M. Interleukins. Available online: https://onlinelibrary.wiley.com/doi/abs/10.1002/9780470015902.a0000932.pub3
- Guo L, Junttila IS, Paul WE. Cytokine-induced cytokine production by conventional and innate lymphoid cells. Trends Immunol 2012;33:598-606. [Crossref] [PubMed]
- Brocker C, Thompson D, Matsumoto A, et al. Evolutionary divergence and functions of the human interleukin (IL) gene family. Hum Genomics 2010;5:30-55. [Crossref] [PubMed]
- Banchereau J, Pascual V, O’Garra A. From IL-2 to IL-37: the expanding spectrum of anti-inflammatory cytokines. Nat Immunol 2012;13:925-31. [Crossref] [PubMed]
- Seruga B, Zhang H, Bernstein LJ, et al. Cytokines and their relationship to the symptoms and outcome of cancer. Nat Rev Cancer 2008;8:887-99. [Crossref] [PubMed]
- Razavi GS, Allen T. Emerging Role of Interleukins in Cancer Treatment. Immunome Res 2014;1.
- Li J, Wang Z, Mao K, et al. Clinical significance of serum T helper 1/T helper 2 cytokine shift in patients with non-small cell lung cancer. Oncol Lett 2014;8:1682-6. [Crossref] [PubMed]
- Gomes M, Coelho A, Araújo A, et al. Influence of functional genetic polymorphism (-590C/T) in non-small cell lung cancer (NSCLC) development: The paradoxal role of IL-4. Gene 2012;504:111-5. [Crossref] [PubMed]
- Cui X, Yang SC, Sharma S, et al. IL-4 regulates COX-2 and PGE2 production in human non-small cell lung cancer. Biochem Biophys Res Commun 2006;343:995-1001. [Crossref] [PubMed]
- Zaynagetdinov R, Sherrill TP, Gleaves LA, et al. Interleukin-5 Facilitates Lung Metastasis by Modulating the Immune Microenvironment. Cancer Res 2015;75:1624-34. [Crossref] [PubMed]
- Chang CH, Hsiao CF, Yeh YM, et al. Circulating interleukin-6 level is a prognostic marker for survival in advanced nonsmall cell lung cancer patients treated with chemotherapy. Int J Cancer 2013;132:1977-85. [Crossref] [PubMed]
- Ujiie H, Tomida M, Akiyama H, et al. Serum hepatocyte growth factor and interleukin-6 are effective prognostic markers for non-small cell lung cancer. Anticancer Res 2012;32:3251-8. [PubMed]
- Gomes M, Coelho A, Araújo A, et al. IL-6 polymorphism in non-small cell lung cancer: a prognostic value? Tumour Biol 2015;36:3679-84. [Crossref] [PubMed]
- Qu Z, Sun F, Zhou J, et al. Interleukin-6 prevents the initiation but enhances the progression of lung cancer. Cancer Res 2015;75:3209-15. [Crossref] [PubMed]
- Li X, Zhang P, Liu X, et al. Expression of interleukin-12 by adipose-derived mesenchymal stem cells for treatment of lung adenocarcinoma. Thorac Cancer 2015;6:80-4. [Crossref] [PubMed]
- Gu K, Li MM, Shen J, et al. Interleukin-17-induced EMT promotes lung cancer cell migration and invasion via NF-κB/ZEB1 signal pathway. Am J Cancer Res 2015;5:1169-79. [PubMed]
- Pan B, Che D, Cao J, et al. Interleukin-17 levels correlate with poor prognosis and vascular endothelial growth factor concentration in the serum of patients with non-small cell lung cancer. Biomarkers 2015;20:232-9. [Crossref] [PubMed]
- Zeng Y, Zhang Q, Wang H, et al. Prognostic significance of interleukin-17 in solid tumors: a meta-analysis. Int J Clin Exp Med 2015;8:10515-36. [PubMed]
- Panneerselvam J, Jin J, Shanker M, et al. IL-24 Inhibits Lung Cancer Cell Migration and Invasion by Disrupting The SDF-1/CXCR4 Signaling Axis. PloS One 2015;10:e0122439 [Crossref] [PubMed]
- Airoldi I, Tupone MG, Esposito S, et al. Interleukin-27 re-educates intratumoral myeloid cells and down-regulates stemness genes in non-small cell lung cancer. Oncotarget 2015;6:3694-708. [Crossref] [PubMed]
- Kim MS, Kim E, Heo JS, et al. Circulating IL-33 level is associated with the progression of lung cancer. Lung Cancer 2015;90:346-51. [Crossref] [PubMed]
- Gu X, Tian T, Zhang B, et al. Elevated plasma interleukin-35 levels predict poor prognosis in patients with non-small cell lung cancer. Tumour Biol 2015;36:2651-6. [Crossref] [PubMed]
- Liao C, Yu ZB, Meng G, et al. Association between Th17-related cytokines and risk of non-small cell lung cancer among patients with or without chronic obstructive pulmonary disease. Cancer 2015;121:3122-9. [Crossref] [PubMed]
- Dmitrieva OS, Shilovskiy IP, Khaitov MR, et al. Interleukins 1 and 6 as Main Mediators of Inflammation and Cancer. Biochemistry (Mosc) 2016;81:80-90. [Crossref] [PubMed]
- Chang Q, Bournazou E, Sansone P, et al. The IL-6/JAK/Stat3 feed-forward loop drives tumorigenesis and metastasis. Neoplasia 2013;15:848-62. [Crossref] [PubMed]
- Tawara K, Oxford JT, Jorcyk CL. Clinical significance of interleukin (IL)-6 in cancer metastasis to bone:Potential of anti-IL-6 therapies. Cancer Manag Res 2011;3:177-89. [PubMed]
- Sun W, Liu DB, Li WW, et al. Interleukin-6 promotes the migration and invasion of nasopharyngeal carcinoma cell lines and upregulates the expression of MMP-2 and MMP-9. Int J Oncol 2014;44:1551-60. [Crossref] [PubMed]
- Zhang X, Yin P, Di D, et al. IL-6 regulates MMP-10 expression via JAK2/STAT3 signaling pathway in a human lung adenocarcinoma cell line. Anticancer Res 2009;29:4497-501. [PubMed]
- Jiang YN, Yan HQ, Huang XB, et al. Interleukin 6 trigged ataxia-telangiectasia mutated activation facilitates lung cancer metastasis via MMP-3/MMP-13 up-regulation. Oncotarget 2015;6:40719-33. [Crossref] [PubMed]
- Xu B, Guenther JF, Pociask DA, et al. Promotion of lung tumor growth by interleukin-17. Am J Physiol Lung Cell Mol Physiol 2014;307:L497-508. [Crossref] [PubMed]
- Zarogoulidis P, Katsikogianni F, Tsiouda T, et al. Interleukin-8 and Interleukin-17 for Cancer. Cancer Invest 2014;32:197-205. [Crossref] [PubMed]
- Wang Y, Wu H, Wu X, et al. Interleukin 17A promotes gastric cancer invasiveness via NF-kB mediated matrix metalloproteinases 2 and 9 expression. PLoS One 2014;9:1-6.
- Zeng Q, Li S, Zhou Y, et al. Interleukin-32 contributes to invasion and metastasis of primary lung adenocarcinoma via NF-kappaB induced matrix metalloproteinases 2 and 9 expression. Cytokine 2014;65:24-32. [Crossref] [PubMed]