TUG1 promotes retinoblastoma progression by sponging miR-516b-5p to upregulate H6PD expression
Introduction
As one of the commonest malignancies in children all over the world, retinoblastoma (RB) has drawn public attention (1). One of every 15,000–20,000 newborns would be diagnosed with RB (2). Though the survival rate of RB patients in developed countries is as high as 95%, the survival rate of them in developing and less developed countries is not more than 70% (3,4). The occurrence of RB is a highly complex process that is closely related to gene mutations (5,6), aberrant expression of oncogenes (7,8), and stimulation of tumorigenic signaling pathways (9,10). The molecular mechanisms of RB development are poorly comprehended. Hence, a better understanding of the potential molecular mechanisms in RB is of considerable merit.
Long non-coding RNAs (lncRNAs) belong to a cluster of non-coding RNAs with over 200 nucleotides in length, lacking in protein-coding ability (11,12). Existing literatures have elucidated that lncRNAs exert pivotal function in the development of multiple cancers. For instance, lncRNA X inactive specific transcript (XIST) sponges miR-140 to facilitate cell proliferation in lung cancer through targeting protein phosphatase 1 regulatory subunit 13 like (iASPP) (13). LncRNA colon cancer associated transcript 1 (CCAT1) functions as a malignancy promoter in melanoma through sponging miR-33a (14). More importantly, lncRNAs are also confirmed to regulate RB tumorigenesis and progression. For instance, lncRNA testis associated oncogenic lncRNA (THOR, also known as THORLNC) promotes RB by motivating the combination of MYC proto-oncogene, bHLH transcription factor (c-myc) with insulin-like growth factor 2 mRNA-binding protein 1 (IGF2BP1) (15). LncRNA H19 imprinted maternally expressed transcript (H19) counteracts miR-17-92 cluster to suppress RB (16). Additionally, lncRNA TUG1, the object of this research, has been revealed to serve as a carcinogenic gene in several cancers (17-19). Nevertheless, its role and regulatory mechanism in RB need more explanations.
Our study took the first endeavor to investigate the function of TUG1 in the occurrence of RB. And we obtained a conclusion that TUG1 sequesters miR-516b-5p to upregulate H6PD, thus promoting cell growth in RB.
Methods
Cell culture and transfection
RB cell lines (Y79, SO-Rb50, WERI-Rb-1 and HXO-Rb44) and normal human retinal capillary endothelial cell line (HRCEC) were bought from the American Type Culture Collection (Shanghai, China). Above cells were cultivated in RPMI-1640 medium (Gibco, Grand Island, NY, USA) containing 10% fetal bovine serum (Gibco) in a moist air of 5% CO2 at 37 °C.
Short hairpin (sh)-TUG1#1/2 was used to knockdown TUG1, and pcDNA3.1/H6PD was used for H6PD overexpression. MiR-516b-5p mimics were applied to overexpress miR-516b-5p. All plasmids were brought from GenePharma (Shanghai, China), which were transfected into WERI-Rb-1 and HXO-Rb44 cells utilizing Lipofectamine 2000 (Invitrogen) based on the manufacturer’s directions.
Real-time quantitative polymerase chain reaction (RT-qPCR) assay
Total RNA was extracted by exploiting TRIzol reagent (Invitrogen, Carlsbad, CA, USA) on the basis of producer’s introductions. Complementary DNA (cDNA) synthesis was carried out by using Pyrobest DNA Polymerase and M-MLV Reverse Transcriptase (Thermo Fisher Scientific, USA) or TaqmanTM Advanced miRNA cDNA Synthesis Kit. PrimeScript RT reagent kit (Takara, DRR037A) was utilized to synthesize the first-strand cDNA, which was amplified by SYBR-Green PCR Master Mix kit (Takara) on an ABI 7500 System (Applied Biosystems, Foster City, CA, USA). Gene expression was calculated by using the 2−ΔΔCt method. Both glyceraldehyde-3-phosphate dehydrogenase (GAPDH) and U6 functioned as internal controls.
Cell counting kit-8 (CCK-8) assay
WERI-Rb-1 and HXO-Rb44 cells transfected with assigned plasmids for one day were put into 96-well plates (1×103 cells/well). After incubation for 0, 24, 48, 72 or 96 h, CCK-8 solution (10 μL) was dropped into each well and transfected cells were subsequently cultured at 37 °C for 4 h. The optical density of each well was estimated at 450 nm by using a microplate reader (EL340; Bio-Tek Instruments, Hopkinton, MA, USA).
Colony formation assay
Cells were laid on 6-well plates with a density of 800 cells per plate. Change the culture medium every 3 days. The cells were then fixed by methanol and dyed with 0.1% crystal violet (Beyotime, Shanghai, China). At last, colonies containing over 50 cells were counted.
5-Ethynyl-2’-Deoxyuridine (EdU) labeling
The EdU incorporation assay was applied in accordance with manufacturer’s instruction as formerly depicted (20).
Caspase-3 activity assay
An APOPCYTO™ Caspase-3 Colorimetric Assay kit (Medical & Biological Laboratories, Co., Ltd., Nagoya, Japan) was employed to estimate caspase-3 activity. In brief, after one day incubation using a colorimetric substrate (DEVD-pNA) at 37 °C, caspase-3 activity was tested by NanoDrop 2000c at an absorbance of 405 nm.
Terminal-deoxynucleoitidyl transferase mediated nick end labeling (TUNEL) assay
The apoptosis of ERI-Rb-1 and HXO-Rb44 cells transfected with different plasmids was assessed by TUNEL analysis as described before (21).
Western blot assay
Total protein was extracted from WERI-Rb-1 and HXO-Rb44 cells utilizing radioimmunoprecipitation assay (RIPA) Lysis Buffer (Beyotime) with protease. After using a BCA Protein Assay kit (Generay, Shanghai, China) to measure the protein concentration, sodium dodecyl sulphate-polyacrylamide gel electrophoresis (SDS-PAGE) was applied for splitting 40 μg of proteins/lane, which were transferred onto polyvinylidene fluoride (PVDF) membranes (Millipore). Five percent non-fatted milk powder was used to block the membranes, which were cultivated with primary antibodies at 4 °C for one night. Then, the membranes were cultured with secondary antibodies at 37 °C for an hour. GAPDH was internal reference.
Subcellular fractionation
Dounce homogenizer was applied for homogenizing 1×105 cells in 0.5 mL of hypotonic suspension buffer, and differential centrifugation was used to separate the cells. The nuclear fraction (P1) was firstly segregated by the centrifugation at 1,000 g and the supernatant was furtherly separated into membrane (P100) and cytosolic (S100) fractions by ultracentrifugation at 100,000 g. One tenth (1×104 cells) of each fraction was exploited to SDS-PAGE and then analyzed.
Luciferase reporter assay
The 3′-untranslated region (UTR) sequences of H6PD which contained the predicted binding sites and full-length sequences of TUG1 were subcloned into the pmirGLO vectors (Promega, Madison, WI, USA), and then the wild type of TUG1/H6PD reporter (TUG1/H6PD-WT) was generated. In a similar way, the mutant type of TUG1/H6PD reporter (TUG1/H6PD-Mut) was produced. The pmirGLO-TUG1/H6PD-WT or pmirGLO-TUG1/H6PD-Mut was co-transfected with miR-516b-5p mimics (or NC mimics) into WERI-Rb-1 and HXO-Rb44 cells using Lipofectamine 2000. And after 2 days, the luciferase activity was assessed by employing luciferase assay kit (Promega Corporation, Fitchburg, WI, USA).
RNA immunoprecipitation (RIP) assay
RIP was exercised utilizing an EZ-Magna RIP RNA-binding protein immunoprecipitation kit (Millipore, Billerica, MA). After 48 h transfection, RIP lysis buffer was utilized to dissolve WERI-Rb-1 and HXO-Rb44 cells. Hundred microliter of cell lysis solution was cultured with RIP buffer involving magnetic beads coated with anti-Ago2 (1:50 dilution; Millipore). IgG (Millipore) served as negative control. Proteinase K buffer was seized to degrade the protein from samples. Subsequently, the extraction and purification of immunoprecipitated RNA were conducted. The expression of TUG11 and miR-516b-5p was detected by RT-qPCR.
Statistical analysis
All analyses were carried out by seizing SPSS for Windows, Version 14.0. (SPSS Inc., Chicago). Each assay was conducted for at least three times. Data were illustrated as mean ± standard deviation (SD). Student’s t-test was adopted to compare the two groups. One-way analysis of variance (ANOVA) was used for multiple groups. P value less than 0.05 was considered of statistical significance.
Results
TUG1 is upregulated in RB cells and TUG1 knockdown inhibits tumorigenesis of RB
To explore the biological function of TUG1 in RB, TUG1 expression in RB cell lines (Y79, SO-Rb50, WERI-Rb-1 and HXO-Rb44) was measured by RT-qPCR analysis, which indicated that TUG1 expression was upregulated in RB cell lines in comparison with normal human retinal capillary endothelial cell line (HRCEC) (Figure 1A). Then, sh-TUG1#1/2 plasmids were transfected into WERI-Rb-1 and HXO-Rb44 cells, and we determined the knockdown efficiency of TUG1 (Figure 1B). Data from CCK-8, colony formation and EdU assays demonstrated that knockdown of TUG1 notably repressed the proliferation of WERI-Rb-1and HXO-Rb44 cells (Figure 1C,D,E). Later, TUNEL and caspase-3 activity analyses manifested that cell apoptosis ability was significantly enhanced after downregulating TUG1 in WERI-Rb-1 and HXO-Rb44 cells (Figure 1F,G). Moreover, TUG1 silence observably decreased Bcl-2 expression while increased the level of Bax and cleaved-caspase 3 in WERI-Rb-1 and HXO-Rb44 cells, and that of total-caspase 3 wasn’t affected (Figure 1H). These data elucidate that TUG1 is upregulated in RB cells and TUG1 knockdown inhibits development of RB.
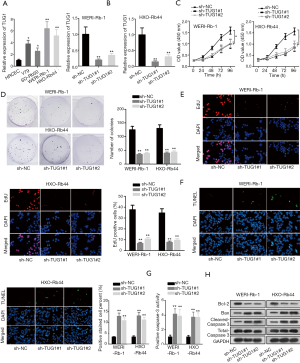
TUG1 sponges miR-516b-5p in RB
To explore whether there are interactions between TUG1 and miRNAs in RB, the following experiments were performed. Nuclear-cytoplasmic fractionation suggested that TUG1 was mainly located in cytoplasm (Figure 2A). As exhibited in Venn diagram, the miRNAs (miR-516b-5p and miR-214-5p) that might bind to TUG1 were screened out through starBase and DIANA public databases (Figure 2B). Then, the expression of miR-516b-5p and miR-214-5p in RB cells was detected. The results uncovered that miR-516b-5p expression was downregulated while miR-214-5p expression was upregulated in RB cell lines compared with HRCEC cells (Figure 2C). Therefore, miR-516b-5p was chosen to conduct the subsequent assays. In addition, we found that TUG1 silence significantly increased miR-516b-5p expression in WERI-Rb-1 and HXO-Rb44 cells (Figure 2D). Besides, RT-qPCR indicated that the increased miR-516b-5p expression was achieved by transfection with miR-516b-5p mimics (Figure 2E). Three binding sites {TargetSite: chr22:31372095-31372117[+], chr22:31371592-31371613[+], chr22:31367507-31367528[+]} in TUG1 for miR-516b-5p were predicted through starBase (Figure 2F). Luciferase reporter assay demonstrated that miR-516b-5p overexpression remarkably decreased the luciferase activity of pmirGLO-TUG1-WT on these three binding sites (Figure 2G). Taken together, TUG1 sponges miR-516b-5p in RB.
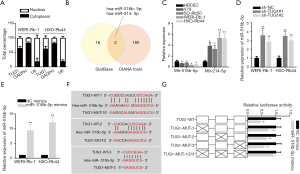
H6PD is targeted by miR-516b-5p in RB
The target gene H6PD was screened out from RNA22, miRmap and microT databases (Figure 3A). RT-qPCR and western blot assays unveiled that H6PD was upregulated in RB cell lines compared with HRCEC cells (Figure 3B). MiR-516b-5p overexpression or TUG1 silence could significantly decrease the expression of H6PD (Figure 3C,D). StarBase predicted that there existed a binding site between miR-516b-5p and H6PD (Figure 3E). Furthermore, luciferase reporter assay verified that miR-516b-5p mimics notably decreased the luciferase activity of pmirGLO-H6PD-WT whereas that of pmirGLO-H6PD-Mut exhibited no evident change in different groups (Figure 3F). Moreover, RIP assay demonstrated that TUG1, miR-516b-5p and H6PD existed in RISC (RNA-induced silencing complex), validated by their obvious enrichments in anti-Ago2 group (Figure 3G). All the results above indicate that H6PD is targeted by miR-516b-5p in RB.
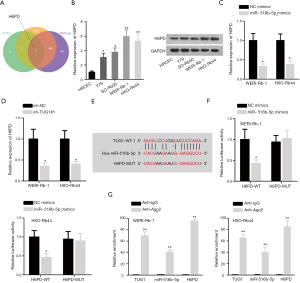
TUG1 promotes the tumorigenesis of RB via modulating H6PD
Finally, to explore whether TUG1 promoted the progression of RB by modulating H6PD expression, several rescue experiments were conducted. Experiments concerning cell proliferation observed that H6PD upregulation partially rescued the inhibitive function of TUG1 knockdown on cell proliferation (Figure 4A,B,C). TUNEL analysis depicted that H6PD upregulation could offset the promoting effect of TUG1 depletion on cell apoptosis (Figure 4D). Western blot assay revealed that H6PD overexpression countervailed the suppressive effects of TUG1 knockdown on the level of anti-apoptosis proteins and stimulating effects of that on the level of pro-apoptosis proteins (Figure 4E). Caspase-3 activity assay displayed that H6PD overexpression neutralized the facilitating function of TUG1 knockdown on cell apoptosis (Figure 4F). All these results suggest that TUG1 promotes the development of RB via upregulating H6PD expression.
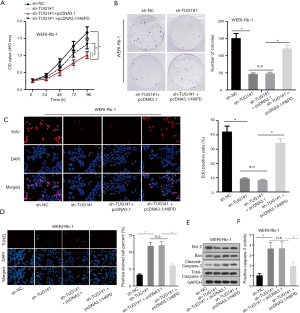
Discussion
Mounting evidences have manifested that lncRNAs exert enormous functions in the occurrence of multiple cancers, like lung cancer, colorectal cancer and melanoma (13,14,22). LncRNA TUG1 has also been recognized as an oncogene in several cancers. For illustration, lncRNA TUG1 promotes the development of papillary thyroid cancer by targeting miR-145 (17). LncRNA TUG1 functions as a malignancy promoter in cervical cancer through the miR-138-5p/sirtuin 1 (SIRT1) axis (19). In our study, TUG1 was upregulated in RB cells and the absence of TUG1 repressed cell proliferation whereas accelerated cell apoptosis in RB. In brief, TUG1 is an oncogenic gene in RB.
As another subtype of non-coding RNAs, microRNAs (miRNAs), possessing a length of 20 to 24 nucleotides, are implicated in the tumorigenesis of multiple cancers (11,23). For instance, miR-509-5p targets MDM2 proto-oncogene (MDM2) to suppress cell metastasis in prostate cancer (24). MiR-223 enhances the progression of breast cancer by inhibiting forkhead box O1 (FOXO1) expression (25). Furthermore, miRNAs have been testified to act as either tumor promoters or suppressors in RB. For example, miR-449a and miR-449b suppress cell proliferation in RB (26). MiR-125a-5p downregulation enhances cell growth in RB through activating the transcriptional co-activator with PDZ binding motif (TAZ)-epidermal growth factor receptor (EGFR) signaling pathway (27). The role of miR-516b-5p had not been investigated in cancers before, and our study discovered that miR-516b-5p was conspicuously downregulated in RB cells and could bind with TUG1.
MiRNAs are also well-known for the regulation of downstream messenger RNA (mRNA) level in abundant cancers. For instance, miR-431 downregulates DEAD-box helicase 5 (DDX5) to inhibit the tumorigenesis in lung cancer (28). MiR-758 targets paired box homeotic gene 6 (PAX6) to serve as a tumor suppressor in RB (29). As an mRNA, H6PD influences cell proliferation and migration in cancers (30). In the current study, H6PD, an upregulated mRNA in RB cells, could bind with miR-516b-5p. And H6PD expression was negatively regulated by miR-516b-5p and positively modulated by TUG1. LncRNAs have been confirmed to competitively bind with specific miRNAs to regulate mRNA, thus affecting the occurrence of cancers. For example, lncRNA H19 sponges miR-138-5p and releases sirtuin-1 (SIRT1) to promote cell growth in cervical cancer (31). LncRNA upregulated in colorectal cancer liver metastasis (UICLM) enhances cell metastasis in colorectal cancer through sponging miR-215 and upregulating zinc finger E-box binding homeobox 2 (ZEB2) (32). Our study demonstrated that the restrained progression of RB induced by TUG1 deficiency was reversed by H6PD overexpression to some extent, indicating that TUG1 facilitated the development of RB via upregulating H6PD.
In a word, our research validated that TUG1 served as a sponge of miR-516b-5p and upregulated H6PD to promote cell growth in RB, possibly inspiring us to find out an effective target for RB treatment.
Acknowledgments
We appreciate the technical supports of the Affiliated Yantai Yuhuangding Hospital of Qingdao University.
Funding: None.
Footnote
Data Sharing Statement: Available at http://dx.doi.org/10.21037/tcr-19-1480
Conflicts of Interest: All authors have completed the ICMJE uniform disclosure form (available at http://dx.doi.org/10.21037/tcr-19-1480). The authors have no conflicts of interest to declare.
Ethical Statement: The authors are accountable for all aspects of the work in ensuring that questions related to the accuracy or integrity of any part of the work are appropriately investigated and resolved.
Open Access Statement: This is an Open Access article distributed in accordance with the Creative Commons Attribution-NonCommercial-NoDerivs 4.0 International License (CC BY-NC-ND 4.0), which permits the non-commercial replication and distribution of the article with the strict proviso that no changes or edits are made and the original work is properly cited (including links to both the formal publication through the relevant DOI and the license). See: https://creativecommons.org/licenses/by-nc-nd/4.0/.
References
- Hu H, Zhang W, Wang Y, et al. Characterization, treatment and prognosis of retinoblastoma with central nervous system metastasis. BMC Ophthalmol 2018;18:107. [Crossref] [PubMed]
- Rao R, Honavar SG. Retinoblastoma. Indian J Pediatr 2017;84:937-44. [Crossref] [PubMed]
- Fabian ID, Onadim Z, Karaa E, et al. The management of retinoblastoma. Oncogene 2018;37:1551-60. [Crossref] [PubMed]
- Jain M, Rojanaporn D, Chawla B, et al. Retinoblastoma in Asia. Eye (Lond) 2019;33:87-96. [Crossref] [PubMed]
- Rojanaporn D, Boontawon T, Chareonsirisuthigul T, et al. Spectrum of germline RB1 mutations and clinical manifestations in retinoblastoma patients from Thailand. Mol Vis 2018;24:778-88. [PubMed]
- Soliman SE, Racher H, Zhang C, et al. Genetics and Molecular Diagnostics in Retinoblastoma--An Update. Asia Pac J Ophthalmol (Phila) 2017;6:197-207. [PubMed]
- Cui Y, Su Y, Deng L, et al. Ginsenoside-Rg5 Inhibits Retinoblastoma Proliferation and Induces Apoptosis through Suppressing BCL2 Expression. Chemotherapy 2018;63:293-300. [Crossref] [PubMed]
- Liu S, Yan G, Zhang J, et al. Knockdown of Long Noncoding RNA (lncRNA) Metastasis-Associated Lung Adeno-carcinoma Transcript 1 (MALAT1) Inhibits Proliferation, Migration, and Invasion and Promotes Apoptosis by Targeting miR-124 in Retinoblastoma. Oncol Res 2018;26:581-91. [Crossref] [PubMed]
- Wang Y, Yuan J, Yang L, et al. Inhibition of migration and invasion by berberine via inactivation of PI3K/Akt and p38 in human retinoblastoma cell line. Adv Clin Exp Med 2018;27:899-905. [Crossref] [PubMed]
- Liao Y, Yin X, Deng Y, et al. MiR-140-5p suppresses retinoblastoma cell growth via inhibiting c-Met/AKT/mTOR pathway. Biosci Rep 2018;38:BSR20180776. [Crossref] [PubMed]
- Zheng Y, Liu L, Shukla GC. A comprehensive review of web-based non-coding RNA resources for cancer research. Cancer Lett 2017;407:1-8. [Crossref] [PubMed]
- Gomes CC, de Sousa SF, Calin GA, et al. The emerging role of long noncoding RNAs in oral cancer. Oral Surg Oral Med Oral Pathol Oral Radiol 2017;123:235-41. [Crossref] [PubMed]
- Tang Y, He R, An J, et al. lncRNA XIST interacts with miR-140 to modulate lung cancer growth by targeting iASPP. Oncol Rep 2017;38:941-8. [Crossref] [PubMed]
- Lv L, Jia JQ, Chen J. The lncRNA CCAT1 Upregulates Proliferation and Invasion in Melanoma Cells via Suppressing miR-33a. Oncol Res 2018;26:201-8. [Crossref] [PubMed]
- Shang Y. LncRNA THOR acts as a retinoblastoma promoter through enhancing the combination of c-myc mRNA and IGF2BP1 protein. Biomed Pharmacother 2018;106:1243-9. [Crossref] [PubMed]
- Wei F, Wu Y, Tang L, et al. BPIFB1 (LPLUNC1) inhibits migration and invasion of nasopharyngeal carcinoma by interacting with VTN and VIM. Br J Cancer 2018;118:233-47. [Crossref] [PubMed]
- Lei H, Gao Y, Xu X. LncRNA TUG1 influences papillary thyroid cancer cell proliferation, migration and EMT for-mation through targeting miR-145. Acta Biochim Biophys Sin (Shanghai) 2017;49:588-97. [Crossref] [PubMed]
- Hu Y, Sun X, Mao C, et al. Upregulation of long noncoding RNA TUG1 promotes cervical cancer cell proliferation and migration. Cancer Med 2017;6:471-82. [Crossref] [PubMed]
- Zhu J, Shi H, Liu H, et al. Long non-coding RNA TUG1 promotes cervical cancer progression by regulating the miR-138-5p-SIRT1 axis. Oncotarget 2017;8:65253-64. [Crossref] [PubMed]
- Song H, Liu Y, Jin X, et al. Long non-coding RNA LINC01535 promotes cervical cancer progression via targeting the miR-214/EZH2 feedback loop. J Cell Mol Med 2019;23:6098-111. [Crossref] [PubMed]
- Cheng T, Xu M, Qin B, et al. lncRNA H19 contributes to oxidative damage repair in the early age-related cataract by regulating miR-29a/TDG axis. J Cell Mol Med 2019;23:6131-9. [Crossref] [PubMed]
- Zhang W, Yuan W, Song J, et al. LncRna CPS1-IT1 Suppresses Cell Proliferation, Invasion and Metastasis in Colorectal Cancer. Cell Physiol Biochem 2017;44:567-80. [Crossref] [PubMed]
- Zhang G, Pian C, Chen Z, et al. Identification of cancer-related miRNA-lncRNA biomarkers using a basic miRNA-lncRNA network. PLoS One 2018;13:e0196681. [Crossref] [PubMed]
- Tian XM, Luo YZ, He P, et al. Inhibition of invasion and migration of prostate cancer cells by miRNA-509-5p via targeting MDM2. Genet Mol Res 2017;16: [Crossref] [PubMed]
- Wei YT, Guo DW, Hou XZ, et al. miRNA-223 suppresses FOXO1 and functions as a potential tumor marker in breast cancer. Cell Mol Biol (Noisy-le-grand) 2017;63:113-8. [Crossref] [PubMed]
- Martin A, Jones A, Bryar PJ, et al. MicroRNAs-449a and -449b exhibit tumor suppressive effects in retinoblastoma. Biochem Biophys Res Commun 2013;440:599-603. [Crossref] [PubMed]
- Zhang Y, Xue C, Zhu X, et al. Suppression of microRNA-125a-5p upregulates the TAZ-EGFR signaling pathway and promotes retinoblastoma proliferation. Cell Signal 2016;28:850-60. [Crossref] [PubMed]
- Xu CM, Chen LX, Gao F, et al. MiR-431 suppresses proliferation and metastasis of lung cancer via down-regulating DDX5. Eur Rev Med Pharmacol Sci 2019;23:699-707. [PubMed]
- Li J, You X. MicroRNA758 inhibits malignant progression of retinoblastoma by directly targeting PAX6. Oncol Rep 2018;40:1777-86. [PubMed]
- Tsachaki M, Mladenovic N, Stambergova H, et al. Hexose-6-phosphate dehydrogenase controls cancer cell proliferation and migration through pleiotropic effects on the unfolded-protein response, calcium homeostasis, and redox balance. FASEB J 2018;32:2690-705. [Crossref] [PubMed]
- Lian Y, Xiong F, Yang L, et al. Long noncoding RNA AFAP1-AS1 acts as a competing endogenous RNA of miR-423-5p to facilitate nasopharyngeal carcinoma metastasis through regulating the Rho/Rac pathway. J Exp Clin Cancer Res 2018;37:253. [Crossref] [PubMed]
- Chen DL, Lu YX, Zhang JX, et al. Long non-coding RNA UICLM promotes colorectal cancer liver metastasis by acting as a ceRNA for microRNA-215 to regulate ZEB2 expression. Theranostics 2017;7:4836-49. [Crossref] [PubMed]