ADRB2 is a potential protective gene in breast cancer by regulating tumor immune microenvironment
Introduction
Breast cancer (BRCA) is by far the most diagnosed and leading cause of cancer death among females. In 2018, it was reported that BRCA accounted for 24.2% of new cancer cases and 15% of cancer deaths among women worldwide (1). According the latest report of cancer statistics in America (2), BRCA provides the greatest number of estimated new cases in 2020, and the incidence rate continues to increase by about 0.5% per year.
After years of in-depth research, the scientific understanding of the crosstalk between immune cells and tumor cells within the tumor microenvironment (TME) has become more comprehensive that the infiltration of immune cells can influence tumor progression and metastasis, as well as therapeutic response (3-5). The arising of immunotherapy targeting PD-1/PD-L1 or CTLA-4 has yielded promising therapeutic fruit in different tumors (6,7). However, quite a few patients cannot benefit from immunotherapy due to resistance, among the reason of which, the insufficient T cells infiltration stands for a significant one (4,8). In recent years, several studies have found that the immune status of BRCA are related to its development (8,9). Some studies demonstrated that BRCA were associated with better prognosis with increased Th1, CD8+ T cells and lower Th2, regulatory T (Treg) cells, Myeloid-derived suppressor cells (MDSCs) (7,8). Therefore, T cells infiltration levels in tumor may be an essential predictive marker for tumor progression and prognosis (5,10).
Clinical studies have suggested that chronic stress or depression and active exercise might influence cancer progression while β-adrenoceptors (β-ARs) could be involved as a major link (11-14). Cells throughout the body express β-ARs, so as cancer cells, from which the β2-adrenergic receptor (β2-AR) take a large part (11,15,16). The gene ADRB2 encodes β2-AR, which is a member of the G protein-coupled receptor superfamily (11,17). It was reported that the two ligands—adrenaline and noradrenaline bind to β-ARs with different affinity, noradrenaline has a higher affinity to β1-AR, and adrenaline to β2-AR (13,18).
As vasoactive drugs, β-ARs agonists are conventionally used for regulating blood pressure cancer patients during anesthetic management. However, it has been shown that β-ARs signaling exerted dual effects in cancers (13,18). Chronic stress led to upregulated MDSCs and Tregs, resulting in immune-suppressive effect, while acute stress and exercise which largely rely on ADRB2 can probably inhibit tumor growth, by leading to an increase of immune cells infiltration in TME. Pedersen et al. (19) showed that exercise enhanced immune cells infiltration across five different tumor models. Studies reported that β2-AR signals perhaps stimulated tumor progression and leaded to poorer prognosis for cancer patients (16,20). For example, Chang et al. found that the ADRB2 on tumor cells played a crucial role in stress-enhanced metastasis in BRCA mouse model (16). Zahalka et al. showed that the loss of endothelial ADRB2 weaken angiogenesis in prostate cancer (20). However, other reports also indicated that β2-AR activation significantly suppressed tumor growth (15,21-24). Sakakitani et al. (24) demonstrated that β2-AR agonist inhibited cell motility and induced mesenchymal-epithelial transition in oral cancer. Moreover, in the research led by Pérez Piñero et al. (25), with the treatment of β-AR agonists, the growth of BRCA cells was significantly inhibited, probably mediated by inhibition of ERK 1/2 phosphorylation. Therefore, the safety and potential risk by using β-ARs agonists in cancer patients need more evidences and discussion.
Here, in this study, with the help of bioinformatic analysis, we investigated the crosstalk between ADRB2 expression and the infiltration of immune cells in BRCA, especially the T cell subtypes. Also, the underlying mechanisms and candidate drugs for treating BRCA were discussed. In sum, ADRB2 is a potential protective gene and a new target for immunotherapy in BRCA. Also, we present the following article in accordance with the MDAR reporting checklist (available at https://dx.doi.org/10.21037/tcr-21-1257).
Methods
Expressions of ADRB2 across The Cancer Genome Atlas (TCGA) database and Kaplan-Meier survival analysis
TCGA is a landmark cancer genomics program which molecularly characterized over 20,000 primary cancer and matched normal samples spanning 33 cancer types. The large sample numbers in TCGA offer an excellent opportunity to address questions associated with tumor heterogeneity. For comparing expressions of ADRB2 across TCGA database, we use UALCAN analysis website (http://ualcan.path.uab.edu) to acquire and analyze expressions of ADRB2 derived from TCGA RNA-seq data in different kinds of cancers and normal tissues (26). Also, expressions of ADRB2 in BRCA based on sample types, individual cancer stages, BRCA subclasses and nodal metastasis status were acquired. Finally, totally 1211 RNA-seq data of BRCA from TCGA dataset was analyzed.
Studies have suggested that cancer could be classified into six different immune subtypes (27): wound healing (C1), IFN-γ dominant (C2), inflammatory (C3), lymphocyte depleted (C4), immunologically quiet (C5), and TGF-β dominant (C6). For better understanding the relationship between ADRB2 and BRCA, associations between ADRB2 expression and immune subtypes in BRCA were analyzed by TISIDB portal (28), which was an integrated repository portal for tumor-immune system interactions (http://cis.hku.hk/TISIDB/). Furthermore, the Kaplan-Meier survival analysis was used to assess the effect of ADRB2 on the overall survival (OS) of BRCA patients, and all survival analyses were performed by using the tool bc-GenExMiner v4.5 (http://bcgenex.ico.unicancer.fr) (29). The optimal cut-off value of ADRB2 was selected and all RNA-seq data (n=4,712) was used for analysis. Log-rank test was used for comparing differences between low/high expression groups.
Expressions of ADRB2 in different normal tissues and blood cells
For comparing the RNA expression levels of ADRB2 in different tissues, relevant data was acquired from the Human Protein Atlas dataset, the Consensus dataset, and the Genotype-Tissue Expression (GTEx) dataset. All data was downloaded from the website of the Human Protein Atlas (HPA, https://www.proteinatlas.org) (30). In the meantime, the RNA expression levels of ADRB2 in different kinds of blood cells were acquired from the Human Protein Atlas dataset, the Consensus dataset, and the Human Blood Atlas dataset (31).
Expression differentiation of ADRB2 in TME of BRCA
Accumulating evidence indicates that the crosstalk between stroma cells and malignant cells within this environment crucially determines the fate of tumor progression. With the aim of exploring the expression differentiation of ADRB2 in TME of BRCA, the authors used a tool named TISCH to enable the exploration of TME in different single-cell transcriptomic analyses of BRCA (http://tisch.comp-genomics.org/home/) (32). Two datasets (and GSE114727_10X) were selected for analyzing the immune infiltration levels (33,34).
Correlations between ADRB2 and immune cell infiltration in BRCA
The correlations between ADRB2 expression and immune cell infiltration in BRCA was analyzed by the TIMER 2.0 database (http://timer.comp-genomics.org/) (35,36). The TIMER 2.0 is a comprehensive resource for systematical analysis of immune infiltrates across diverse cancer types. It provides immune infiltrates’ abundances estimated by multiple immune deconvolution methods, and allows users to generate high-quality figures dynamically to explore tumor immunological, clinical and genomic features comprehensively. The correlations among gene expression levels and immune cell infiltration levels (CD4+ T cell, CD8+ T cell, gamma delta T cell (Tγδ), follicular helper T cell (Tfh), Treg, NK T cell, B cell, myeloid dendritic cell, NK cell, neutrophil, macrophage, monocyte, endothelial cell and MDSC) were acquired from the TIMER 2.0. Also, the correlations among T cell subtypes (CD4+ T cell, CD8+ T cell, Tfh and Treg) and ADRB2 copy number variations (CNVs) were detected in the TIMER 2.0 database.
Co-expression genes analysis and functional enrichment analyses
The co-expression genes of ADRB2 in BRCA were analyzed by using the cBio Cancer Genomics Portal (cBioPortal, http://cbioportal.org) (37). The cBioPortal is an open-access resource for interactive exploration of multidimensional cancer genomics data sets, and provides access to data from more than 30,000 tumor samples from 334 cancer studies. Also, the co-expression scatter plots among ADRB2 and immune cell-related marker genes were obtained from the cBioPortal. The Breast Invasive Carcinoma (TCGA, Firehose Legacy) dataset in cBioPortal was selected for analyses.
For understanding the underlying mechanism of ADRB2 in BRCA, the gene ontology (GO) enrichment analyses and KEGG pathways analyses were performed. The co-expression genes which Spearman’s correlation score >0.45 were selected for functional enrichment analyses, and the top20 most enriched pathways were shown in figures. All functional enrichment analyses were performed using the Sangerbox tools, a free online platform for data analysis (http://www.sangerbox.com/tool).
Constructing protein-protein interaction (PPI) networks of ADRB2 in BRCA
For understanding the potential PPI among ADRB2 and co-expression genes, the authors constructed a PPI network using the Search Tool for the Retrieval of Interacting Genes (STRING) online database (http://version10.string-db.org/) (38). The top 300 co-expression genes (ranked by the Spearman’s correlation score) were selected for PPI analysis. Interactions which interaction score <0.9 and genes which had no direct/indirect interactions with ADRB2 would be hidden.
Transcription factor (TF) targets and regulatory TFs prediction
To explore the potential TF targets of ADRB2 and predict TFs which regulate the ADRB2, gene enrichments analyses of Transcription-Factor-Targets and TRRUST were identified by using the Metascape website (http://metascape.org/) (39). The Metascape was a web-based portal and provided a comprehensive gene list annotation and analysis resource for researchers, and combined gene annotation, functional enrichment, interactome analysis and membership search to leverage over 40 independent knowledgebases within one integrated portal.
Potential targeted drugs prediction and interactions between chemicals and ADRB2
To predict interactions between known chemicals and ADRB2, the STITCH version 5.0 database was used (http://stitch.embl.de/) (40). Also, Connectivity Map database (CMap, https://clue.io/, data version: 1.1.1.2) was used to identify small molecular candidate drugs related to BRCA treatments (41). The CMap is a collection of genome-wide transcriptional expression data from cultured human cells treated with bioactive small molecules, and it can help researchers discover the functional connections between drugs, genes and diseases through the transitory feature of common gene-expression changes. By inputting positive co-expressed genes and negative co-expressed genes of ADRB2 in CMap, we could acquire candidate drugs which resulted in similar gene changes in cancer cells. The top 10 potential targeted drugs in BRCA cell lines were acquired (ranked by the correlation score).
Statistical analysis
Expressions of ADRB2 were generated by the UALCAN and HPA website. Kaplan-Meier survival curves were generated by the tool bc-GenExMiner v4.5, and the log-rank t-test was used to compare differences between two groups. The correlation between ADRB2 expression and immune cell infiltration was analyzed by the TIMER 2.0 database, and regression curves were drawn. The co-expression genes of ADRB2 in BRCA were analyzed by using the cBioPortal and scatter diagrams were shown in log scale. Also, the Spearman’ correlation scores and Pearson scores were calculated. Non-parametric tests followed by the Kruskal-Wallis test was utilized for multiple groups comparisons. For functional enrichment analyses, significant enrichment pathways with q-value<0.1 and P value <0.05 were shown. Small molecular drug candidates were identified by using CMap database. P values <0.05 were considered statistically significant.
Ethical statement
The study was conducted in accordance with the Declaration of Helsinki (as revised in 2013).
Results
Expressions of ADRB2 were significantly down-regulated and leaded to worse prognosis in BRCA
According to expressions of ADRB2 across TCGA cancers, it was common that expressions of ADRB2 were significantly decreased in multiple cancer types included BRCA, bladder cancer, cholangiocarcinoma, colon adenocarcinoma, lung adenocarcinoma, lung squamous cell carcinoma, etc. (Figure 1A,1B). Further analyses suggested that ADRB2 expressions were also down-regulated in any cancer stages, BRCA subclasses and nodal metastasis status (Figure 1C-1E). Moreover, we found no obvious differences in different cancer stages (except stage 1 vs. stage 2) and nodal metastasis status.
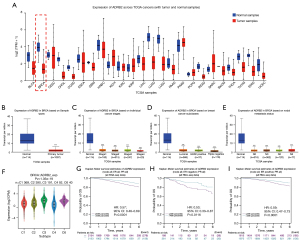
According to recent studies, tumors could be identified as six immune subtypes (C1-C6), and C3 subtype often indicated the best prognosis, while C4, C5 and C6 leaded to the worst prognosis. Also, C2 showed the highest lymphocyte infiltration and CD8+ signal, while C4 the opposite. In BRCA, we found that the mean expression of ADRB2 in C4 was the lowest while in C3 was the highest in five immune subtypes (Figure 1F). Therefore, we reckoned that ADRB2 connected with the immune function tightly. Next, survival analyses showed that higher level of ADRB2 predicted better prognosis (Figure 1G-1I and Table S1). In conclusion, these findings revealed that ADRB2 was distinctly down-regulated in BRCA, and lower level of ADRB2 linked with worse prognosis in patients.
Expression and distribution heterogeneity of ADRB2 in tissues and immune cells
By analyzing expressions of ADRB2 in different tissues in three public datasets, we found that ADRB2 was mainly expressed in breast, skin, blood and adipose tissue (Figure 2A-2C). Especially in the blood, the ADRB2 was highly expressed in diverse immune cells (Figure 2A,2B). After comparing expressions of ADRB2 in different immune cells, we found that ADRB2 was mainly presented in T cell subtypes, except for Treg (Figure 2D-2F). It demonstrated again that ADRB2 might participate in regulating immune responses and T cells tumor infiltration.
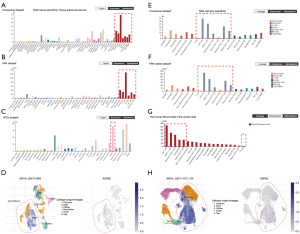
Next, we verified this finding in two single-cell transcriptomic analyses of BRCA (GSE110686 and GSE114727_10X). Both of them revealed that (Figure 2G,2H), in TME of BRCA, ADRB2 mainly presented in CD8+ T cells and CD4+ Tconv (CD4+CD25- T cells). Collectively, these results suggested that the ADRB2 might regulate TME of BRCA by targeting T cells.
The expression level and CNVs of ADRB2 determined the immune cell infiltration in BRCA
Correlations between ADRB2 and immune cell infiltration in BRCA were analyzed. As shown in Figure 3A, ADRB2 expression level was positively correlated with the infiltration levels of CD4+ T cell, CD8+ T cell, Tγδ, myeloid DC, neutrophil, macrophage, monocyte and endothelial cell. On the contrary, ADRB2 expression level was negatively correlated with the infiltration levels of immune-suppressed cell subtypes such as Treg, Tfh and MDSC. It suggested that more immune-activated cell subtypes infiltrated into TME in BRCA patients with high levels of ADRB2. Also, CNVs analyses of ADRB2 revealed that the infiltration levels of CD4+ T cell and CD8+ T cell were higher in normal, gain and high amplification patients, while in deletion patients, Tfh constituted a higher portion (Figure 3B). No difference was found between CNVs and Treg infiltration level.
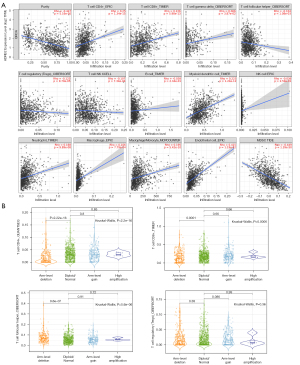
Co-expression genes functional enrichment analyses and PPI networks construction of ADRB2 in BRCA
Co-expression genes of ADRB2 in BRCA were acquired from the cBioPortal, and genes with Spearman’s correlation score >0.45 were listed in Table S2. Functional enrichment analyses of GO (including biological process, cellular component and molecular function) and KEGG signal pathways were displayed in Figure 4A-4D and Figure S1. Results showed that most enriched pathways were immune-related no matter in KEGG or GO enrichment analyses, particularly for T cell-related signal pathways. For example, in top20 GO-biological process enrichment analysis, T cell activation ranked as the most significantly enriched pathway. Also, almost all pathways in top20 GO-biological process enrichment analysis were immune-related. It strongly suggested that the ADRB2 might play a vital role in immune responses of BRCA.
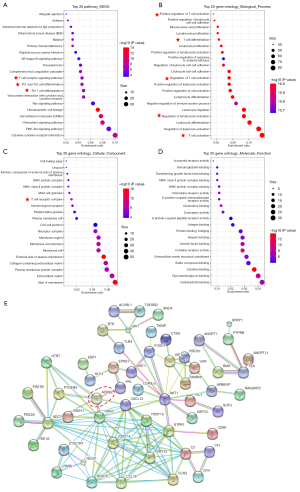
The potential PPI among ADRB2 and co-expression genes showed that ADRB2 was correlated with many immune-related genes such as CXCL12, CCR2, CCL19, FOXO1 and C3. Also, AKT signal pathway might interacted with the ADRB2 tightly (Figure 4E).
Co-expression scatter plots among ADRB2 and immune cell-related marker genes
Immune cell-related marker genes were listed in Table S3. By analyzing scatter plots among the expression of ADRB2 and immune cell-related marker genes, we found that higher expression levels of ADRB2 often correlated with higher levels of immune cell-related marker genes (Figure 5). Especially for CD3, CD4, CD8 and STAT4, the Spearman scores exceeded 0.4. Interestingly, Treg related marker genes were also positively correlated with the ADRB2. Nevertheless, the Spearman scores and Pearson scores were relatively lower than other immune cell-related marker genes.
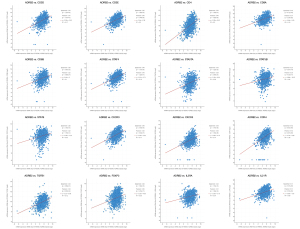
Potential regulatory mechanism and targeted drugs prediction
The potential downstream TFs regulated by ADRB2 were showed in Figure 6A, and most of these TFs were immune-related and regulated immune function such as MAML1, ELF1, STAT6, STAT5B, STAT4, etc. Also, TFs which might regulate expression of ADRB2 were listed in Figure 6B, such as NF-κB family, RFX family and GATA family.
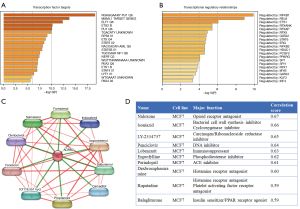
Known chemicals which targeted to ADRB2 were showed in Figure 6C, such as salmeterol, salbutamol and formoterol. According to our studies, we predicted that drugs which activated ADRB2 might be beneficial for immune infiltration and brought better prognosis in BRCA. Furthermore, the top10 small molecular drug candidates for BRCA were listed in Figure 6D. These candidate drugs involved in opioid receptor antagonist, carcinogen inhibitor, DNA inhibitor, histamine receptor inhibitors, etc. Studies had suggested that these candidate drugs showed promising effects on inhibiting tumor progression such as naloxone (42), isoniazid (43), LY-2334737 (44) (also known as the oral gemcitabine) and penciclovir (Figure S2). These results further supported our hypothesis that ADRB2 was a potential protective gene in BRCA, and drugs which activated ADRB2 might be beneficial for the prognosis of BRCA patients.
Discussion
In this comprehensive and multiple-layer bioinformatic analysis, we found a new protective gene named ADRB2 which significantly influenced the prognosis of BRCA patients. Our findings showed that ADRB2 was mainly expressed in breast and immune cells especially in T cell subtypes, and single-cell transcriptomic analyses of BRCA revealed that almost all ADRB2 was presented in CD8+ T cells and CD4+ Tconv in TME. Further functional enrichment analyses suggested that most enriched pathways were immune-related. Regression analyses between ADRB2 and co-expression genes indicated higher expression levels of ADRB2 often correlated with higher levels of immune cell-related marker genes. Finally, downstream TFs prediction demonstrated that most of these TFs regulated by ADRB2 were immune-related. These results strongly suggested that ADRB2 might play a vital role in immune infiltration of BRCA.
For centuries, a large number of studies had suggested that somatic and psychosocial factors may significantly affect cancer development (11,13,14,17,18). However, the underlying mechanisms of psychosocial factors were still not elucidated with more detail. Among diverse psychosocial factors, interactions of stress, hypothalamic-pituitary-adrenal (HPA) axis and sympathetic nervous system (SNS) were one of the hottest areas. Studies revealed that stress would cause physiological distress and result in an abnormal release of hormones, which elevated expression of oncogenes and induced numerous cancer biological processes, such as angiogenesis, proliferation, metastasis, metabolic disorders and immune inhibition (13,19,45). These effects were mainly mediated via β-ARs, especially the β2-AR subtype. Moreover, clinical trials aiming to investigate the clinical value of β-blockers (antagonists of β2-AR) showed signs of clinical potential in improving prognosis of cancer patients (45-47).
However, studies also suggested that acute stress helped the body to regain homeostasis (18,19,48). For example, there were strong data in support of a positive impact of exercise on cancer incidence and OS in cancer patients (19,49). Mouse models also showed that adrenaline, secreted in association with exercise, could activate immune cells and lead to an increase in numbers of immune cells infiltrating into tumors, resulting in therapeutic efficacy (19,49). Strikingly, the effects on cell mobilization, tumor influx of immune cells and therapeutic impact would be reversed by blocking the β2-AR. Consequently, it was hard to clarify the actual effects of β-ARs on cancer development based on existing data.
In clinical anesthesia, β2-AR agonists are commonly used for balancing blood pressure for cancer patients. Whether β2-AR agonists application will influence the development of cancer is of high clinical value. Some studies have shown that the activation of β2-AR was associated with the deterioration of several types of cancers such as BRCA, pancreatic cancer, gastric cancer and ovarian cancer (16,21,47,50,51). The underlying mechanisms including the enhancement cell proliferation, migration and invasion, tumor angiogenesis and immune evasion by activating the β2-AR signals. On the contrary, numerous studies concluded distinct results in terms of the β2-AR signals. Bravo-Calderón et al. (22) showed that 10 µM of norepinephrine (a β2-AR agonist) significantly inhibited the migration of SCC-9 and SCC-25 oral squamous cell carcinoma cell lines while a significant reversion was observed by using β2-AR antagonist propranolol. Baker et al. (52) found that acute systemic β-AR activation in healthy donors markedly augmented the mobilization, expansion, and anti-tumor activity of Tγδ cells. In spontaneous model of mammary adenocarcinoma, Dawes et al. (12) found that stress exposure significantly suppressed tumor growth, but not metastasis. The stress-induced decrease in tumor burden was associated with increased apoptosis within the tumor and reduced frequency of immunosuppressive MDSCs in lung and spleen, and decreased circulating exosome TGF-β2. Furthermore, stress-induced tumor inhibition was reversed by nadolol (a non-selective β-AR antagonist that could not cross the blood-brain barrier), indicating that the tumor inhibitory effects of stress relied on peripheral β-AR signaling. These results were consistent with our findings that ADRB2 expression level was positively correlated with the infiltration levels of Tγδ cells while negatively correlated with the MDSCs.
Though a number of studies held the view that ADRB2 accelerated the progression and metastasis of BRCA, supporting evidences regarding the protective effects of ADRB2 were also verified in BRCA. In a clinical study, Caparica et al. (15) found that high ADRB2 expression might be favorable in patients with HER2+ early BRCA, and ADRB2 was associated with a low expression of angiogenesis-related and proliferation-related genes; and a high expression of immune-related genes. These findings were consisted with our results that higher expression levels of ADRB2 correlated with higher expression levels of immune cell-related marker genes. Similarly, in another clinical study (53), researchers found that, in HER2+ BRCA patients, strong β2-AR expression correlated with better disease-free survival. In our survival analyses, similar protective effects of ADRB2 were also observed (Figure 1 and Table S1). Fundamental studies revealed that β2-AR activation inhibited the proliferation and metastasis of BRCA. Gargiulo et al. (54) found that β2-AR knock-down caused a significant increase of cell proliferation and migration, and a decrease of cell adhesion in BRCA cell lines. Rivero et al. (23) showed that salbutamol (β2-agonist) significantly reduced migration and invasion of BRCA cell lines while epinephrine exerted opposite effects. Therefore, ADRB2 might be a protective gene in BRCA.
Relationships among stress, SNS and cancer are complex and controversies are remained. The discrepancy may be associated with the specificity of cancer types, organs, and tissues. Also, the length and intensity of stress, the activation of different AR subtypes and the combined effects of different signal pathways, hormones and immune responses would lead to opposite results (55-57). For example, by analyzing 1924 BRCA patients’ Affymetrix gene expression datasets, Rivero et al. observed a significant increase in distant metastasis-free survival of patients with high ADRA2A and ADRB2 while a decrease with ADRA2C high expression (56). Different cancer cell lines responded oppositely in β-AR signals. Madden et al. (55) found that β-AR activation increased cAMP level in MCF7 and MB-361 much less than in MB-231 and MB-231BR (BRCA cell lines). Moreover, β2-AR stimulation did not markedly alter vascular endothelial growth factor (VEGF) production in MCF7 or MB-361 while decrease VEGF production by MB-231 and increase VEGF production in MB-231BR.
Some limitations of the present study are worth noting. First, in the next step, fundamental studies vitro and vivo are needed to demonstrate our findings. Also, some prospective or experimental clinical validations should be performed to verify the effect of ADRB2 in BRCA patients. Second, regulations between ADRB2 and immune infiltration should be detected in BRCA mouse models, and the top enriched pathways should be verified as well. Third, it would be helpful to clarify effects of ADRB2 on BRCA patients by carrying out a randomized controlled trial related to β2-AR agonists.
Conclusions
In conclusion, ADRB2 was a potential protective gene in BRCA, and it might play a vital role in regulating immune responses and immune infiltration in TME. The expression level of ADRB2 was positively correlated with immune cells infiltration in BRCA, especially for T cells. Therefore, ADRB2 would be a target for boosting immunotherapy effects in BRCA.
Acknowledgments
We would like to thank professor Christian Weber for his help in polishing our paper.
Funding: This study is supported by the Pyramid Talented Personnel Project of Changzheng Hospital (awarded to Doctor Chengxiang Shan).
Footnote
Reporting Checklist: The authors have completed the MDAR reporting checklist. Available at https://dx.doi.org/10.21037/tcr-21-1257
Conflicts of Interest: All authors have completed the ICMJE uniform disclosure form (available at https://dx.doi.org/10.21037/tcr-21-1257). The authors have no conflicts of interest to declare.
Ethical Statement: The authors are accountable for all aspects of the work in ensuring that questions related to the accuracy or integrity of any part of the work are appropriately investigated and resolved. The study was conducted in accordance with the Declaration of Helsinki (as revised in 2013).
Open Access Statement: This is an Open Access article distributed in accordance with the Creative Commons Attribution-NonCommercial-NoDerivs 4.0 International License (CC BY-NC-ND 4.0), which permits the non-commercial replication and distribution of the article with the strict proviso that no changes or edits are made and the original work is properly cited (including links to both the formal publication through the relevant DOI and the license). See: https://creativecommons.org/licenses/by-nc-nd/4.0/.
References
- Bray F, Ferlay J, Soerjomataram I, et al. Global cancer statistics 2018: GLOBOCAN estimates of incidence and mortality worldwide for 36 cancers in 185 countries. CA Cancer J Clin 2018;68:394-424. [Crossref] [PubMed]
- Siegel RL, Miller KD, Fuchs HE, et al. Cancer Statistics, 2021. CA Cancer J Clin 2021;71:7-33. [Crossref] [PubMed]
- Hegde PS, Chen DS. Top 10 Challenges in Cancer Immunotherapy. Immunity 2020;52:17-35. [Crossref] [PubMed]
- Tabana Y, Okoye IS, Siraki A, et al. Tackling Immune Targets for Breast Cancer: Beyond PD-1/PD-L1 Axis. Front Oncol 2021;11:628138. [Crossref] [PubMed]
- van der Leun AM, Thommen DS, Schumacher TN. CD8+ T cell states in human cancer: insights from single-cell analysis. Nat Rev Cancer 2020;20:218-32. [Crossref] [PubMed]
- Havel JJ, Chowell D, Chan TA. The evolving landscape of biomarkers for checkpoint inhibitor immunotherapy. Nat Rev Cancer 2019;19:133-50. [Crossref] [PubMed]
- Kalbasi A, Ribas A. Tumour-intrinsic resistance to immune checkpoint blockade. Nat Rev Immunol 2020;20:25-39. [Crossref] [PubMed]
- Jenkins S, Wesolowski R, Gatti-Mays ME. Improving Breast Cancer Responses to Immunotherapy-a Search for the Achilles Heel of the Tumor Microenvironment. Curr Oncol Rep 2021;23:55. [Crossref] [PubMed]
- Agostinetto E, Eiger D, Punie K, et al. Emerging Therapeutics for Patients with Triple-Negative Breast Cancer. Curr Oncol Rep 2021;23:57. [Crossref] [PubMed]
- Fiore D, Cappelli LV, Broccoli A, et al. Peripheral T cell lymphomas: from the bench to the clinic. Nat Rev Cancer 2020;20:323-42. [Crossref] [PubMed]
- Cui B, Peng F, Lu J, et al. Cancer and stress: NextGen strategies. Brain Behav Immun 2021;93:368-83. [Crossref] [PubMed]
- Dawes RP, Burke KA, Byun DK, et al. Chronic Stress Exposure Suppresses Mammary Tumor Growth and Reduces Circulating Exosome TGF-β Content via β-Adrenergic Receptor Signaling in MMTV-PyMT Mice. Breast Cancer (Auckl) 2020;14:1178223420931511. [Crossref] [PubMed]
- Iftikhar A, Islam M, Shepherd S, et al. Cancer and Stress: Does It Make a Difference to the Patient When These Two Challenges Collide? Cancers (Basel) 2021;13:163. [Crossref] [PubMed]
- Kamiya A, Hiyama T, Fujimura A, et al. Sympathetic and parasympathetic innervation in cancer: therapeutic implications. Clin Auton Res 2021;31:165-78. [Crossref] [PubMed]
- Caparica R, Richard F, Brandão M, et al. Prognostic and Predictive Impact of Beta-2 Adrenergic Receptor Expression in HER2-Positive Breast Cancer. Clin Breast Cancer 2020;20:262-273.e7. [Crossref] [PubMed]
- Chang A, Le CP, Walker AK, et al. β2-Adrenoceptors on tumor cells play a critical role in stress-enhanced metastasis in a mouse model of breast cancer. Brain Behav Immun 2016;57:106-15. [Crossref] [PubMed]
- Conceição F, Sousa DM, Paredes J, et al. Sympathetic activity in breast cancer and metastasis: partners in crime. Bone Res 2021;9:9. [Crossref] [PubMed]
- Jensen AWP, Carnaz Simões AM, Thor Straten P, et al. Adrenergic Signaling in Immunotherapy of Cancer: Friend or Foe? Cancers (Basel) 2021;13:394. [Crossref] [PubMed]
- Pedersen L, Idorn M, Olofsson GH, et al. Voluntary Running Suppresses Tumor Growth through Epinephrine- and IL-6-Dependent NK Cell Mobilization and Redistribution. Cell Metab 2016;23:554-62. [Crossref] [PubMed]
- Zahalka AH, Arnal-Estapé A, Maryanovich M, et al. Adrenergic nerves activate an angio-metabolic switch in prostate cancer. Science 2017;358:321-6. [Crossref] [PubMed]
- Albiñana V, Gallardo-Vara E, de Rojas-P I, et al. Targeting β2-Adrenergic Receptors Shows Therapeutical Benefits in Clear Cell Renal Cell Carcinoma from Von Hippel-Lindau Disease. J Clin Med 2020;9:2740. [Crossref]
- Bravo-Calderón DM, Assao A, Garcia NG, et al. Beta adrenergic receptor activation inhibits oral cancer migration and invasiveness. Arch Oral Biol 2020;118:104865. [Crossref] [PubMed]
- Rivero EM, Piñero CP, Gargiulo L, et al. The β 2-Adrenergic Agonist Salbutamol Inhibits Migration, Invasion and Metastasis of the Human Breast Cancer MDA-MB- 231 Cell Line. Curr Cancer Drug Targets 2017;17:756-66. [Crossref] [PubMed]
- Sakakitani S, Podyma-Inoue KA, Takayama R, et al. Activation of β2-adrenergic receptor signals suppresses mesenchymal phenotypes of oral squamous cell carcinoma cells. Cancer Sci 2021;112:155-67. [Crossref] [PubMed]
- Pérez Piñero C, Bruzzone A, Sarappa MG, et al. Involvement of α2- and β2-adrenoceptors on breast cancer cell proliferation and tumour growth regulation. Br J Pharmacol 2012;166:721-36. [Crossref] [PubMed]
- Chandrashekar DS, Bashel B, Balasubramanya SAH, et al. UALCAN: A Portal for Facilitating Tumor Subgroup Gene Expression and Survival Analyses. Neoplasia 2017;19:649-58. [Crossref] [PubMed]
- Thorsson V, Gibbs DL, Brown SD, et al. The Immune Landscape of Cancer. Immunity 2018;48:812-830.e14. [Crossref] [PubMed]
- Ru B, Wong CN, Tong Y, et al. TISIDB: an integrated repository portal for tumor-immune system interactions. Bioinformatics 2019;35:4200-2. [Crossref] [PubMed]
- Jézéquel P, Gouraud W, Ben Azzouz F, et al. bc-GenExMiner 4.5: new mining module computes breast cancer differential gene expression analyses. Database (Oxford) 2021;2021:baab007.
- Sjöstedt E, Zhong W, Fagerberg L, et al. An atlas of the protein-coding genes in the human, pig, and mouse brain. Science 2020;367:eaay5947. [Crossref] [PubMed]
- Uhlen M, Karlsson MJ, Zhong W, et al. A genome-wide transcriptomic analysis of protein-coding genes in human blood cells. Science 2019;366:eaax9198. [Crossref] [PubMed]
- Sun D, Wang J, Han Y, et al. TISCH: a comprehensive web resource enabling interactive single-cell transcriptome visualization of tumor microenvironment. Nucleic Acids Res 2021;49:D1420-30. [Crossref] [PubMed]
- Azizi E, Carr AJ, Plitas G, et al. Single-Cell Map of Diverse Immune Phenotypes in the Breast Tumor Microenvironment. Cell 2018;174:1293-1308.e36. [Crossref] [PubMed]
- Savas P, Virassamy B, Ye C, et al. Single-cell profiling of breast cancer T cells reveals a tissue-resident memory subset associated with improved prognosis. Nat Med 2018;24:986-93. [Crossref] [PubMed]
- Li T, Fu J, Zeng Z, et al. TIMER2.0 for analysis of tumor-infiltrating immune cells. Nucleic Acids Res 2020;48:W509-14. [Crossref] [PubMed]
- Newman AM, Liu CL, Green MR, et al. Robust enumeration of cell subsets from tissue expression profiles. Nat Methods 2015;12:453-7. [Crossref] [PubMed]
- Gao J, Aksoy BA, Dogrusoz U, et al. Integrative analysis of complex cancer genomics and clinical profiles using the cBioPortal. Sci Signal 2013;6:pl1. [Crossref] [PubMed]
- Szklarczyk D, Franceschini A, Wyder S, et al. STRING v10: protein-protein interaction networks, integrated over the tree of life. Nucleic Acids Res 2015;43:D447-52. [Crossref] [PubMed]
- Zhou Y, Zhou B, Pache L, et al. Metascape provides a biologist-oriented resource for the analysis of systems-level datasets. Nat Commun 2019;10:1523. [Crossref] [PubMed]
- Szklarczyk D, Santos A, von Mering C, et al. STITCH 5: augmenting protein-chemical interaction networks with tissue and affinity data. Nucleic Acids Res 2016;44:D380-4. [Crossref] [PubMed]
- Subramanian A, Narayan R, Corsello SM, et al. A Next Generation Connectivity Map: L1000 Platform and the First 1,000,000 Profiles. Cell 2017;171:1437-1452.e17. [Crossref] [PubMed]
- Katakami N, Harada T, Murata T, et al. Randomized Phase III and Extension Studies of Naldemedine in Patients With Opioid-Induced Constipation and Cancer. J Clin Oncol 2017;35:3859-66. [Crossref] [PubMed]
- Yang XG, Li YF, Wang YJ, et al. Application of novel pH sensitive isoniazid-heptamethine carbocyanine dye conjugates against prostate cancer cells. Pharmazie 2020;75:412-6. [PubMed]
- Pratt SE, Durland-Busbice S, Shepard RL, et al. Human carboxylesterase-2 hydrolyzes the prodrug of gemcitabine (LY2334737) and confers prodrug sensitivity to cancer cells. Clin Cancer Res 2013;19:1159-68. [Crossref] [PubMed]
- Mravec B, Horvathova L, Hunakova L. Neurobiology of Cancer: the Role of β-Adrenergic Receptor Signaling in Various Tumor Environments. Int J Mol Sci 2020;21:7958. [Crossref] [PubMed]
- Liu D, Zha L, Liu Y, et al. β2-AR activation promotes cleavage and nuclear translocation of Her2 and metastatic potential of cancer cells. Cancer Sci 2020;111:4417-28. [Crossref] [PubMed]
- Zhang C, Liao X, Ma Z, et al. Overexpression of β-Adrenergic Receptors and the Suppressive Effect of β2-Adrenergic Receptor Blockade in Oral Squamous Cell Carcinoma. J Oral Maxillofac Surg 2020;78:1871.e1-1871.e23. [Crossref]
- Turner JE, Brum PC. Does Regular Exercise Counter T Cell Immunosenescence Reducing the Risk of Developing Cancer and Promoting Successful Treatment of Malignancies? Oxid Med Cell Longev 2017;2017:4234765. [Crossref] [PubMed]
- Bucsek MJ, Qiao G, MacDonald CR, et al. β-Adrenergic Signaling in Mice Housed at Standard Temperatures Suppresses an Effector Phenotype in CD8+ T Cells and Undermines Checkpoint Inhibitor Therapy. Cancer Res 2017;77:5639-51. [Crossref] [PubMed]
- Chang CH, Lee CH, Ko JC, et al. Effect of β-Blocker in Treatment-Naïve Patients With Advanced Lung Adenocarcinoma Receiving First-Generation EGFR-TKIs. Front Oncol 2020;10:583529. [Crossref] [PubMed]
- Zhang X, Zhang Y, He Z, et al. Chronic stress promotes gastric cancer progression and metastasis: an essential role for ADRB2. Cell Death Dis 2019;10:788. [Crossref] [PubMed]
- Baker FL, Bigley AB, Agha NH, et al. Systemic β-Adrenergic Receptor Activation Augments the ex vivo Expansion and Anti-Tumor Activity of Vγ9Vδ2 T-Cells. Front Immunol 2020;10:3082. [Crossref] [PubMed]
- Du Y, Zhou L, Wang Y, et al. Association of alpha2a and beta2 adrenoceptor expression with clinical outcome in breast cancer. Curr Med Res Opin 2014;30:1337-44. [Crossref] [PubMed]
- Gargiulo L, Copsel S, Rivero EM, et al. Differential β2-adrenergic receptor expression defines the phenotype of non-tumorigenic and malignant human breast cell lines. Oncotarget 2014;5:10058-69. [Crossref] [PubMed]
- Madden KS, Szpunar MJ, Brown EB. β-Adrenergic receptors (β-AR) regulate VEGF and IL-6 production by divergent pathways in high β-AR-expressing breast cancer cell lines. Breast Cancer Res Treat 2011;130:747-58. [Crossref] [PubMed]
- Rivero EM, Martinez LM, Bruque CD, et al. Prognostic significance of α- and β2-adrenoceptor gene expression in breast cancer patients. Br J Clin Pharmacol 2019;85:2143-54. [Crossref] [PubMed]
- Wang J, Zhang X, Li J, et al. ADRB1 was identified as a potential biomarker for breast cancer by the co-analysis of tumor mutational burden and immune infiltration. Aging (Albany NY) 2020;13:351-63. [Crossref] [PubMed]