Emerging evidence for the role of an endorectal balloon in prostate radiation therapy
Introduction
External beam radiation treatment is a well-established treatment modality for prostate cancer patients. Advancements in radiation treatment such as three-dimensional conformal radiation therapy (3DCRT), intensity-modulated radiotherapy (IMRT), and proton therapy (PT) have allowed for highly conformal dose distribution to the target and consequently improved normal tissue sparing when dose escalation was performed. Radiation doses greater than 70 Gy have demonstrated greater local tumor control and improved biochemical outcomes, therefore delivery of higher doses of radiation has been attempted in order to further improve outcomes (1,2). Although a direct relationship between the level of dose administered and outcome has been shown, dose escalation in prostate cancer radiation therapy (RT) was traditionally limited by the associated rectal toxicities.
Due to prostate motion and setup uncertainties and to avoid significant deviation from the prescribed dose, planning target volume (PTV) margins are applied to the clinical target volume (CTV) to ensure dosimetric coverage of the prostate (3). The CTV-to-PTV margin may also increase the risk of irradiating surrounding normal tissues such as the rectum and may lead to increased anorectal toxicity. The impact of PTV margins may be even more dramatic in the setting of stereotactic and hypofractionated prostate RT (4). Therefore, reducing the internal margin (IM) is critical in order to further escalate dose while the rate of rectum complications are maintained within clinically acceptable limits.
Particular to proton RT, the PTV also has to account for the range uncertainty present along the proton beam direction (5) and therefore, a beam orientation dependent PTV is defined. The traditional beam arrangement clinically employed in proton RT in prostate cancer has historically varied, from the perineal boost field (6), to parallel opposed, or slightly angled opposed fields (7). However, this paradigm may change if anteriorly oriented beams would be employed, as suggested by Tang et al. (8). Based on this work, the endorectal balloon (ERB), Figure 1, may have a particularly relevant role in range verification, required to ensure the safe delivery of daily proton RT utilizing this anterior beam arrangement.
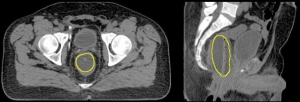
An extensive literature review conducted by Smeenk et al. (9) demonstrated that the role of the ERB in prostate RT to improve prostate fixation, rectal sparing, and outcome is not entirely clear despite more than three decades since it was first described in the literature by Shipley et al. (6). The presumption is that the ERBs ability to reduce prostate intrafraction motion would allow for improved target localization, tighter IM, and decreased anorectal toxicity.
In order to clarify the role of a daily-ERB in prostate RT, Smeenk et al. identified the need for prospective clinical studies using real-time and imaging surveillance for position verification and methods to reduce gas and stool volumes (9). As the use of ERBs expands, the need to reexamine its role in prostate RT becomes even more critical. A PubMed/MEDLINE search using the keywords: ERB, prostate RT, toxicities, real-time, IGRT, RGRT, and interfraction and intrafraction prostate motion was performed in order to identify new publications related to the use of daily-ERB for prostate RT since the review of Smeenk et al. (9). Ten prospective and retrospective ERB studies have since been published and form the basis of this review (8,10-18).
The issue of motion
Four of the ten studies pertain to the issue of prostate motion and are reviewed below (19-22). Radiofrequency-guided radiotherapy (RGRT) and image-guided radiation therapy (IGRT) have allowed for the use of advanced conformal RT techniques by monitoring interfraction and intrafraction tumor motion during treatment. IGRT utilizes specialized imaging such as a computed tomography (CT) scan, cone beam CT (CBCT) scan, ultrasound, or X-rays as a means to improve dose delivery. Technologies such as cine-magnetic resonance imaging (MRI), CT, and Calypso tracking systems (Calypso Medical Technologies Inc., Seattle, WA) have examined interfraction and intrafraction prostate motion, offline or online, relative to the patient treatment delivery. Calypso is the only in-beam real time tracking system which has been extensively used in the clinical setting for RGRT (19,20). Real-time prostate tracking during treatment delivery provides further insight into the role of daily-ERB in prostate intrafraction motion management.
Motion has been a critical factor during advanced prostate RT and was previously, generally managed using external and internal immobilization devices. External anatomical variations are minimized by positioning the patient in a secure and reproducible manner based on indexed immobilization devices such as Knee-Lok and Foot-Lok cushions or personalized Vac-Lok body casts (CIVCO, Orange City, IA). Internal prostate fixation may be achieved using an air- or water-filled daily-ERB. Besides the external and internal immobilization devices, IGRT largely used in modern RT has been widely applied to prostate alignment based on implanted fiducial or prostate 3D representation.
Patient preparation for prostate RT may be particularly important as the prostate resides between organs with variable volumes such as the rectum and bladder. Intrafraction motion significantly hinders target localization as a result from rectal peristalsis, distention, and respiration (21,22). Studies using cine-MRI of non-ERB patient suggested that prostate motion is mostly due to the effect of gas pockets in the filled rectum (23).
The effect of stool and gas pockets on motion may affect the ERBs ability to reduce random intrafraction motions (10). Some advocate for patients’ pretreatment bladder and bowel preparations, such as daily use of anti-gas tablets with meals beginning 1-2 weeks prior, laxative suppositories or enemas within 1-4 hours prior, and voiding followed by immediately drinking adequate fluid (approximately 16 ounces of water) to achieve full bladder filling 20-30 min prior to CT simulation. Recently, Wootton et al. retrospectively examined the effectiveness of an ERB with passive gas release conduit on the removal of rectal gas for prostate proton radiotherapy. Two groups of fifteen patients treated with standard ERB and gas-release ERB were analyzed based on lateral kilovoltage (KV) images and the results showed that the mean incidence of gas in the anterior and other regions differed at a statistically significant level (11). Although the main limitation of this study was the lack of volumetric data, the possibility to identify the gas anterior to the ERB based on the lateral KV for a large number of fractions allowed the authors to conclude in favor of the use of the gas-release ERB for patients undergoing proton RT which mostly occurs with two parallel opposed beams. A potential advantage of a daily-ERB is that it allows for standardization of the rectal volume to minimize the daily variability of the prostate position, leading to improved target localization over the course of treatment (12).
As mentioned previously, 4 of the 10 recent reports have evaluated the effect of ERB on reducing prostate intrafraction motion via RGRT (10,12-14). Both et al. (13) prospectively studied real-time prostate intrafraction motion as a function of treatment time to determine an optimal IM for ERB patients and addressed the patient-specific intrafraction motion. A daily-ERB (RadiaDyne, LLC, Houston, TX) was filled with 100 cc of water. All patients received 79.2 Gy to the PTV in 44 fractions of 1.8 Gy per fraction via IMRT or Varian RapidArc (Varian2300IX; Varian Medical Systems, Palo Alto, CA). The balloon position relative to the rectum canal was ensured through the use of an indexed ERB, positioned based on the value obtained at the time of simulation. Calypso tracking system was utilized to evaluate three-dimensional (3D), lateral (L), cranial-caudal (CC), and anterior-posterior (AP) displacements for a group of 24 patients with a total of 787 tracking sessions. The average percentage of time with 3D, L, CC, and AP prostate displacements >2, 3, 4, 5, 6, 7, 8, 9, and 10 mm in 1 minute intervals was calculated for up to 6 minutes of treatment time. 3D analysis showed that prostate motion is dependent on treatment time for displacements >2, 3, and 4 mm. Interestingly, displacements >5 mm showed time-independence, and the larger motions >6 mm were negligible within 6 minutes treatment time. The overall average time with prostate displacement >3 mm was 5%, suggesting that a 3 mm IM would sufficiently cover 95% of the treatment time within a 6 minute interval. Moreover, for treatment times longer than 6 minutes, a 5 mm IM may be considered to cover more than 95% of time due to the time-independence of the motion >5 mm observed. Directional analysis shown in this study illustrated negligible lateral prostate motion while the AP and CC motions were comparable. The authors also indicated that no obvious relationship exists between the percentage of time at displacement and the week of treatment course, indicating the use of an ERB obviates the correlation between bowel habit changes and rectal volume over the treatment course. Their findings suggested that use of a daily-ERB consistently stabilized prostate motion and prevented clinically significant displacements to occur.
Following the study of Both et al. (13), Wang et al. (14) further compared the intrafraction motion between 30 (1,008 sessions) ERB and 29 (1,061 sessions) non-ERB patients groups. The same patient preparations described by Both et al. (13) were applied to both groups. Large 3D motion (up to 1 cm or more) was noted in the non-ERB group. The motion increased as a function of time for displacements >2-8 mm for the non-ERB group and >2-4 mm for the ERB group. The authors also indicated that the percentage time distributions between the two groups were significantly different for motion >5 mm. The 3D symmetrical IM can be reduced by 40% from 5 to 3 mm if an ERB is chosen as the internal immobilization device. Based on the similar directional analysis as described in Both et al. (13), this study showed that the percentage of time the prostate displaced in any direction was less in the ERB group than the non-ERB group, with a particularly large motion reduction shown in the anterior-posterior directions, which may allow for dose escalation while sparing surrounding normal tissues such as bladder and rectum (4,24). The motion patterns of the patients representing the worst-case scenario for both groups were analyzed in this study, which found that the percentage time of prostate displacements >3 up to 10 mm was consistently higher for the non-ERB patient group.
Smeenk et al. (10) investigated prostate intrafraction motion during RT and performed a one-to-one comparison of 15 ERB (567 sessions) to non-ERB patients (576 sessions). All patients received a total dose of 80 Gy in 2 Gy fractions and the ERB patients were applied with a 100 cc air-filled balloon (QLRAD B.V., Dalfsen, Netherlands). The intrafraction motions were analyzed in 150 second timeframes, using the Calypso system, at displacements >1, 3, 5, and 7 mm for 3D vector analysis to determine where motion was most volatile. The analysis showed displacements <5 mm were more frequent for both groups. However, after 150 seconds there was a linear increase of displacement with time, most notably for displacements >3 mm. There were significantly smaller variances of the percentages of 3D displacement >3, 5, and 7 mm when treated with an ERB. Intrafraction motion of 3D-vector deviations >1, 3, 5, and 7 mm were 57.7%, 7.0%, 0.7%, and 0.3% in the ERB group vs. 70.2%, 18.1%, 4.6%, and 1.4% in the non-ERB group after 10 minutes. Prostate interfraction motion was evaluated and they found insignificant interfraction variation between cohorts with and without a daily-ERB but there were significantly less intrafraction motions with an ERB (10,15). The data suggested a 5 mm IM to be sufficient for prostate intrafraction motion when using an ERB (10), as similarly indicated by Both et al. and Wang et al.
Hung et al. (12) investigated daily interfraction prostate motion comparing two cohorts of patients (14/15) treated with fiducial markers implanted in the prostate with and without daily-ERB. Based on portal imaging, the daily displacements necessary to place the prostate at the isocenter were determined and analyzed. The change in interfraction prostate motion over the course of treatment was reduced for the ERB group, however not statistically significant and therefore the use of daily image guidance was still recommended when daily-ERBs are employed.
Dosimetric studies
Two of the 10 recent reports have assessed the dosimetric consequences and potential benefit of ERB use. In the first, Smeenk et al. (15) investigated the dosimetric effect of the ERB on anorectal toxicities post-prostatectomy IMRT for 20 patients who underwent salvage IMRT treatment planning with a prescribed dose of 70 Gy with and without a 100 cc air-filled ERB (QLRAD B.V., Dalfsen, Netherlands). Comparative analysis reported significant reductions of the anal wall (Awall) dose-volume indicators except for V70Gy, while the mean dose was reduced by an average of 6 Gy. The rectal wall (Rwall) V30, V40, and A40 were found to be significantly reduced as well. According to this dosimetric study the use of an ERB has the potential to spare the anorectal wall and in particular the Awall in high-dose post-prostatectomy IMRT.
In the second dosimetric study, Tang et al. (8) conducted a detailed dosimetric comparison among anterior, anterior-oblique, and lateral passive scattering proton beams for 10 patients treated with a daily-ERB has shown that the anterior-oriented beams can fully exploit the sharp distal penumbra to spare the rectum and provide superior dose distribution. The rectal volume that receives 95% of the prescription dose in the anterior beams is about 1/10 of that in the lateral beams. The mean dose of rectum and penile bulb can also be reduced by about a factor of two. Femoral heads are not included in the anterior-oriented beams and hence received negligible dose but the bladder received a much higher dose in the anterior beams. However, an optimal plan can be produced to significantly reduce the rectal dose without compromising the bladder dose by properly weighting all the available beams. In addition, the introduction of anterior-oriented fields allows for the possibility of either reducing treatment toxicity at current prescription doses or further dose escalation in the treatment of prostate cancer.
In order to correct for range uncertainty due to bladder volume variability when anterior beams are employed an array of dosimeters can be placed on the anterior surface of the ERB for the purpose of range verification as well as dose monitoring during treatment (8). An in vivo range verification method particularly for the passive scattering delivery system has shown millimeter accuracy in phantom tests (25,26). With a small amount of dose from a probing beam delivered to the detectors at the beginning of the treatment, the residual range of the probing beam can be determined, which is then used to adjust the treatment beam. The pretreatment “range check” using detectors placed on the ERB makes the anterior-oriented proton beams clinically feasible and offer the ability to deliver improved dose distributions in proton prostate RT.
Clinical outcomes
The Smeenk et al. review (9) summarized the GI toxicity outcomes of patients treated with an ERB during radiotherapy for the potential benefit of prostate fixation, dose escalation, and anorectal sparing, as reviewed in Table 1, specifically for photon therapy. Since then 3 additional reports discussed below have examined clinical outcomes (20-22) in photon therapy. The first, also noted in Table 1, has reported clinical outcomes in terms of acute GI and GU toxicities using a water-filled ERB during IMRT (16).
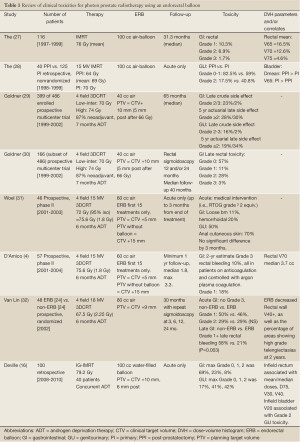
Full table
Prostate proton therapy is delivered in conjunction with a water-filled daily-ERB to eliminate the dose heterogeneities in the beam. In contrast, photon radiation therapy reports indicate most commonly the use of air-filled ERBs for the benefit of anterior rectal wall sparing at the risk of diminished posterior target coverage. Song et al. (33) reviewed conventional treatment planning to address heterogeneity by comparing the dose calculations to a Monte Carlo simulation using the four-field box technique and found that the treatment planning system inferred higher dose regions resulting in potential under dosage of 3.4% mean dose for the posterior beam near the peripheral zone of the prostate, where up to 74% of the prostate cancer foci are located (34). Thus, a water-filled ERB, has more recently been employed during IMRT to reduce dose heterogeneity and potential dose calculation errors due to treatment planning algorithm limitations which could lead to diminished target coverage.
Deville et al. (16) reported the acute GI and GU toxicity rates for 100 prostate cancer patients undergoing image-guided intensity-modulated radiation therapy (IG-IMRT) with a daily endorectal water-filled balloon (ERBH2O), using an indexed-lumen 100 cc ERBH2O to 79.2 Gy in 1.8 Gy fractions. They found that Grade ≥2 GI and GU toxicity rates of 8% and 42%, respectively, compared favorably with (I) patients treated with IMRT using an ERBair - for which there is only single institution data from the Baylor group, reporting rates of acute GI and GU toxicity of 18% and 35%, respectively, in 396 prostate cancer patients treated from 1997-2001 with mean dose 77 Gy (specifically 70 Gy in 2 Gy daily fractions prescribed to the 85% isodose line) IMRT using 100 cc ERBair (35) - and (II) with the more extensively reported acute GI and GU toxicity rates for non-ERB prostate IMRT including their own institution at 13% and 50%, respectively (36).
In an in-depth analysis of anorectal toxicity, Smeenk et al., recently investigated the relationship between anal and rectal DVH parameters and GI incontinence-related complaints such as urgency, incontinence, and frequency in 60 prostate cancer patients undergoing external beam RT (3- or 4-field 3DCRT or 5-field IMRT to 67.5 or 70 Gy in 2.25 or 2.5 Gy fractions) between 2000-2007 using anorectal manometry and barostat measurements to evaluate anal pressures, rectal capacity, and rectal sensory functions at least 90 days post-treatment (17). Half were treated with an 80 cc air-filled ERB. They found that in patients with (I) frequency - almost all rectal parameters were reduced, (II) urgency - several anal wall and rectal wall were predictive, such as the anal Dmean >38 Gy, and (III) incontinence - some anal wall parameters correlated. Patients treated with an ERB described significantly fewer complaints than patients treated without a balloon, which was therefore attributed to receiving lower doses to the Awall and Rwall.
In a related report, Smeenk et al., retrospectively investigated the relationship between fecal incontinence-related complaints and individual pelvic floor muscles (the internal anal sphincter (IAS) muscle, the external anal sphincter (EAS) muscle, the puborectalis muscle (PRM), and the levator ani muscles (LAM), in addition to the Awall and Rwall in 48 patients undergoing prostate radiotherapy (3- or 4-field 3DCRT or 5-field IMRT to 67.5 or 70 Gy in 2.25 or 2.5 Gy fractions), 28 patients with an 80 or 100 cc air-filled ERB (18). They found that urgency was associated with several anal and rectal wall parameters, as well as doses to all separate pelvic floor muscles, while incontinence was associated mainly with doses to the EAS and PRM. Based on the dose-effect curves, they suggested the following mean doses to reduce the risk of urgency: ≤30 Gy to the IAS; ≤10 Gy to the EAS; ≤50 Gy to the PRM; and ≤40 Gy to the LAM. Finally, similar to the previous study, they found that patients treated with an ERB reported significantly less urgency and incontinence, attributed to significantly lower doses to the Awall, Rwall, and all pelvic floor muscles.
Conclusions
The emerging evidence of the role of ERB in prostate RT consist mainly of real-time tracking of the prostate motion with and without an ERB and showed a favorable reduction in the IM required when a daily-ERB was employed while the introduction of gas release ERBs seems promising. Dosimetric studies suggest improved dose distributions when the ERB is employed using parallel opposed beams and especially for anteriorly oriented beams with ERB guided range verification. The outcome study of Deville et al. presents promising finding for early GI toxicity with a water-filled ERB, however late toxicity data should be awaited. Correlative studies of late rectal function and anorectal dosimetry by Smeenk et al. provided clinical evidence for the dosimetric gains noted with an ERB. Further investigation of SV variation and involvement, rectal deformation, and stool and air contributions are merited and will likely comprise future directions.
Acknowledgments
Funding: None.
Footnote
Provenance and Peer Review: This article was commissioned by the Guest Editors (Huan Giap and Eric Y Chuang) for the series “Particle Beam Therapy I” published in Translational Cancer Research. The article has undergone external peer review.
Conflicts of Interest: All authors have completed the ICMJE uniform disclosure form (available at http://dx.doi.org/10.3978/j.issn.2218-676X.2012.10.10). The series “Particle Beam Therapy I” was commissioned by the editorial office without any funding or sponsorship. The authors have no other conflicts of interest to declare.
Ethical Statement: The authors are accountable for all aspects of the work in ensuring that questions related to the accuracy or integrity of any part of the work are appropriately investigated and resolved.
Open Access Statement: This is an Open Access article distributed in accordance with the Creative Commons Attribution-NonCommercial-NoDerivs 4.0 International License (CC BY-NC-ND 4.0), which permits the non-commercial replication and distribution of the article with the strict proviso that no changes or edits are made and the original work is properly cited (including links to both the formal publication through the relevant DOI and the license). See: https://creativecommons.org/licenses/by-nc-nd/4.0/.
References
- King CR, Spiotto MT. Improved outcomes with higher doses for salvage radiotherapy after prostatectomy. Int J Radiat Oncol Biol Phys 2008;71:23-7. [PubMed]
- Zelefsky MJ, Kollmeier M, Cox B, et al. Improved clinical outcomes with high-dose image guided radiotherapy compared with non-IGRT for the treatment of clinically localized prostate cancer. Int J Radiat Oncol Biol Phys 2012;84:125-9. [PubMed]
- ICRU Report 62. Prescribing, Recording and Reporting Photon Beam Therapy (Supplement to ICRU Report 50). Bethesda: ICRU, 1999:ix+52.
- D’Amico AV, Manola J, McMahon E, et al. A prospective evaluation of rectal bleeding after dose-escalated three-dimensional conformal radiation therapy using an intrarectal balloon for prostate gland localization and immobilization. Urology 2006;67:780-4. [PubMed]
- Report ICRU. 78. Prescribing, Recording, and Reporting Proton-Beam Therapy. International Commission on Radiation Units and Measurements 2007;7:1473-6691.
- Shipley WU, Tepper JE, Prout GR Jr, et al. Proton radiation as boost therapy for localized prostatic carcinoma. JAMA 1979;241:1912-5. [PubMed]
- DeLaney TF, Kooy HM. eds. Proton and charged particle radiotherapy. Philadelphia: Lippincott Williams & Wilkins, 2008.
- Tang S, Both S, Bentefour H, et al. Improvement of prostate treatment by anterior proton fields. Int J Radiat Oncol Biol Phys 2012;83:408-18. [PubMed]
- Smeenk RJ, Teh BS, Butler EB, et al. Is there a role for endorectal balloons in prostate radiotherapy? A systematic review. Radiother Oncol 2010;95:277-82. [PubMed]
- Smeenk RJ, Louwe RJ, Langen KM, et al. An endorectal balloon reduces intrafraction prostate motion during radiotherapy. Int J Radiat Oncol Biol Phys 2012;83:661-9. [PubMed]
- Wootton LS, Kudchadker RJ, Beddar AS, et al. Effectiveness of a novel gas-release endorectal balloon in the removal of rectal gas for prostate proton radiation therapy. J Appl Clin Med Phys 2012;13:3945. [PubMed]
- Hung AY, Garzotto M, Kaurin D. Minimal benefit of an endorectal balloon for prostate immobilization as verified by daily localization. Med Dosim 2011;36:195-9. [PubMed]
- Both S, Wang KK, Plastaras JP, et al. Real-time study of prostate intrafraction motion during external beam radiotherapy with daily endorectal balloon. Int J Radiat Oncol Biol Phys 2011;81:1302-9. [PubMed]
- Wang KK, Vapiwala N, Deville C, et al. A study to quantify the effectiveness of daily endorectal balloon for prostate intrafraction motion management. Int J Radiat Oncol Biol Phys 2012;83:1055-63. [PubMed]
- Smeenk RJ, Louwe RJ, Langen KM, et al. An endorectal balloon reduces intrafraction prostate motion during radiotherapy. Int J Radiat Oncol Biol Phys 2012;83:661-9. [PubMed]
- Deville C, Both S, Bui V, et al. Acute gastrointestinal and genitourinary toxicity of image-guided intensity modulated radiation therapy for prostate cancer using a daily water-filled endorectal balloon. Radiat Oncol 2012;7:76. [PubMed]
- Smeenk RJ, Hopman WP, Hoffmann AL, et al. Differences in radiation dosimetry and anorectal function testing imply that anorectal symptoms may arise from different anatomic substrates. Int J Radiat Oncol Biol Phys 2012;82:145-52. [PubMed]
- Smeenk RJ, Hoffmann AL, Hopman WP, et al. Dose-effect relationships for individual pelvic floor muscles and anorectal complaints after prostate radiotherapy. Int J Radiat Oncol Biol Phys 2012;83:636-44. [PubMed]
- Kupelian P, Willoughby T, Mahadevan A, et al. Multi-institutional clinical experience with the Calypso System in localization and continuous, real-time monitoring of the prostate gland during external radiotherapy. Int J Radiat Oncol Biol Phys 2007;67:1088-98. [PubMed]
- Willoughby TR, Kupelian PA, Pouliot J, et al. Target localization and real-time tracking using the Calypso 4D localization system in patients with localized prostate cancer. Int J Radiat Oncol Biol Phys 2006;65:528-34. [PubMed]
- Beard CJ, Kijewski P, Bussière M, et al. Analysis of prostate and seminal vesicle motion: implications for treatment planning. Int J Radiat Oncol Biol Phys 1996;34:451-8. [PubMed]
- Little DJ, Dong L, Levy LB, et al. Use of portal images and BAT ultrasonography to measure setup error and organ motion for prostate IMRT: implications for treatment margins. Int J Radiat Oncol Biol Phys 2003;56:1218-24. [PubMed]
- Ghilezan MJ, Jaffray DA, Siewerdsen JH, et al. Prostate gland motion assessed with cine-magnetic resonance imaging (cine-MRI). Int J Radiat Oncol Biol Phys 2005;62:406-17. [PubMed]
- Smeenk RJ, van Lin EN, van Kollenburg P, et al. Anal wall sparing effect of an endorectal balloon in 3D conformal and intensity-modulated prostate radiotherapy. Radiother Oncol 2009;93:131-6. [PubMed]
- Lu HM. A potential method for in vivo range verification in proton therapy treatment. Phys Med Biol 2008;53:1413-24. [PubMed]
- Bentefour el H. Effect of tissue heterogeneity on an in vivo range verification technique for proton therapy. Phys Med Biol 2012;57:5473-84. [PubMed]
- Teh BS, Dong L, McGary JE, et al. Rectal wall sparing by dosimetric effect of rectal balloon used during intensity-modulated radiation therapy (IMRT) for prostate cancer. Med Dosim 2005;30:25-30. [PubMed]
- Teh BS, Mai WY, Augspurger ME, et al. Intensity modulated radiation therapy (IMRT) following prostatectomy: more favorable acute genitourinary toxicity profile compared to primary IMRT for prostate cancer. Int J Radiat Oncol Biol Phys 2001;49:465-72. [PubMed]
- Goldner G, Bombosch V, Geinitz H, et al. Moderate risk-adapted dose escalation with three-dimensional conformal radiotherapy of localized prostate cancer from 70 to 74 Gy. First report on 5-year morbidity and biochemical control from a prospective Austrian-German multicenter phase II trial. Strahlenther Onkol 2009;185:94-100. [PubMed]
- Goldner G, Tomicek B, Becker G, et al. Proctitis after external-beam radiotherapy for prostate cancer classified by Vienna Rectoscopy Score and correlated with EORTC/RTOG score for late rectal toxicity: results of a prospective multicenter study of 166 patients. Int J Radiat Oncol Biol Phys 2007;67:78-83. [PubMed]
- Woel R, Beard C, Chen MH, et al. Acute gastrointestinal, genitourinary, and dermatological toxicity during dose-escalated 3D-conformal radiation therapy (3DCRT) using an intrarectal balloon for prostate gland localization and immobilization. Int J Radiat Oncol Biol Phys 2005;62:392-6. [PubMed]
- van Lin EN, Kristinsson J, Philippens ME, et al. Reduced late rectal mucosal changes after prostate three-dimensional conformal radiotherapy with endorectal balloon as observed in repeated endoscopy. Int J Radiat Oncol Biol Phys 2007;67:799-811. [PubMed]
- Song JS, Court LE, Cormack RA. Monte Carlo calculation of rectal dose when using an intrarectal balloon during prostate radiation therapy. Med Dosim 2007;32:151-6. [PubMed]
- Chen ME, Johnston DA, Tang K, et al. Detailed mapping of prostate carcinoma foci: biopsy strategy implications. Cancer 2000;89:1800-9. [PubMed]
- Bastasch MD, Teh BS, Mai WY, et al. Tolerance of endorectal balloon in 396 patients treated with intensity-modulated radiation therapy (IMRT) for prostate cancer. Am J Clin Oncol 2006;29:8-11. [PubMed]
- Deville C, Both S, Bui V, et al. Acute gastrointestinal and genitourinary toxicity of image-guided intensity modulated radiation therapy for prostate cancer using a daily water-filled endorectal balloon. Radiat Oncol 2012;7:76. [PubMed]