ECSIT inhibits cell death to increase tumor progression and metastasis via p53 in human breast cancer
Introduction
Cancer is a major public health problem worldwide, and breast cancer is the second leading cause of cancer deaths in women (1). Based on GLOBOCAN, in 2020, female breast cancer became the most commonly diagnosed cancer with an incidence of 11.7% of total cancer cases (2). According to the pathogenesis of breast cancer, different types have different therapeutic targets. BRCA1/2, TP53, CDH1, PTEN, HER2, ER, PR and STK11 were biomarkers of breast cancer (3), the mutation of these genes related to the risk of breast cancer. For hormone receptor-positive breast cancer, hormone therapy can significantly improve the 5-year survival rate of patients (4). However, this is a long-term process, and the effect is not obvious in the short term. HER2, as a target in breast cancer, can effectively kill tumors through the combination of drugs, but there is a negative effect, that may lead to drug resistance and poor prognosis (5). The loss of PTEN can upregulate the expression of PD-L1 in triple-negative breast cancer (TNBC) cells, providing a new therapeutic basis for targeting PD-L1 (6). TROP2 also has a negative effect as a therapeutic target for TNBC (7). In a word, some of these biomarkers were already applied in clinical diagnosis and treatment, however the death rates for breast cancer were still high. We need to look for new molecular targets to improve their effectiveness in reducing the incidence and mortality of breast cancer.
Complicated signaling pathways have been related in the proliferation, invasion, migration, and metastasis of breast cancer. The goal of current breast cancer therapies is destruction of cancer cells, which rely heavily on the ability of a cancer cell to engage its own cell death program. Furthermore, metastatic cells also need to suppress cell death following detachment from the trophic environment and extracellular matrix (8). However, it is not clear whether breast cancer has signaling pathways or new key molecules that are intrinsically associated with cell death.
Previous studies indicate that constitutive activation of nuclear factor-κB (NF-κB), which drives the expression of genes important for inflammation, immunity, cell migration and cell survival, plays a major role in tumor development. It is also found in most tumor types, including leukemia, lymphomas and solid tumors (9,10). Recent studies have shown that downregulation of NF-κB p65 inhibits the development of ovarian cancer (11) and that upregulation of PAK5 promotes breast cancer progression by increasing the nuclear translocation of p65 (12). ECSIT (an evolutionarily conserved signaling intermediate in Toll pathways) is an important intermediate in the TLR/IL-1R signal transduction pathway that is required for NF-κB activation (13). Targeting null mutation of ECSIT leads to reduced cell proliferation, impaired mesoderm formation and embryonic lethality on embryonic Day 7.5 (E7.5) (14). Notably, ECSIT interacts specifically with the multiadaptor protein and E3 ubiquitin ligase—tumor necrosis factor receptor-associated factor (TRAF) 6 (15). The inhibition of TRAF6 ubiquitin-ligase activity could attenuate the ubiquitination of ECSIT, which is essential for NF-κB activation and autophagy activation after TLR4 stimulation (16). In addition, an NF-κB interacting lncRNA (NKILA) interacts with NF-κB/IκB, thereby inhibiting NF-κB activation and suppressing cancer metastasis (17). Evidence shows that NF-κB is frequently activated in breast cancer and that inhibition of NF-κB activity suppresses breast cancer progression (18,19). These findings support the therapeutic exploitation of cell death and NF-κB as a new approach for cancer therapy.
Usually, the level of the tumor suppressor p53 is low or undetectable. Activated p53 induces apoptosis and cellcycle arrest to protect tumor development. p53 also regulates cell apoptosis by the caspase signaling pathway and activates the expression of genes that inhibit survival signaling (20). p53 and NF-κB generally have opposing effects in cancer cells. The transcription factor NF-κB shows strong anti-apoptotic activity in many systems by inhibiting cell death (21). However, the role of NF-κB in modulating p53-induced apoptosis remains unclear. No connection between ECSIT and p53 and no evidence showing its role in breast cancer have been reported. We present the following article in accordance with the MDAR reporting checklist (available at https://tcr.amegroups.com/article/view/10.21037/tcr-21-2234/rc).
Methods
Cell culture
The MDA-MB-231 and 293T cell lines (obtained from the Cell Bank of the Shanghai Chinese Academy of Sciences in March 2016 and October 2014) were grown in Dulbecco’s modified Eagle’s medium (DMEM, HyClone, China), each supplemented with 10% heat-inactivated fetal bovine serum (Biological Industries, Kibbutz Beit Haemek, Israel) and 100 U/mL penicillin/streptomycin (Beyotime, China). The cells were authenticated by STR profiling. Cells were collected for use when they were in the logarithmic growth phase. The study was conducted in accordance with the Declaration of Helsinki (as revised in 2013).
Generation of stable cell lines
For ECIST knockdown, Lipofectamine 3000 (Invitrogen, USA) was used to transfect a MISSION short-hairpin RNA (shRNA) targeting human ECSIT (shECSIT) or MISSION nontargeting random shRNA (shRandom) (Sigma-Aldrich, USA) into 293T cells.
In the presence of polybrene (8 µg/mL), MDA-MB-231 cells were infected with pseudoviral particles and selected with puromycin (2 µg/mL) for 48 h to select stable virus infected cells.
Plasmids and cell transfection
According to the manufacturer’s instructions, plasmids were transfected into cells using Lipofectamine® 3000 transfection reagent (Invitrogen, USA) in serum-free Opti-MEM (Gibco). An empty vector was used as a negative control.
We developed small interfering RNA (siRNA) to suppress the expression of p53 in shECSIT breast cancer cells, and it was constructed and generated by GenePharma (Shanghai, China). The target sense sequence was: 5’-ATGGATCCGTGACACGCTTCCCTGGATTG-3’.
Cell proliferation assay
The proliferative activity of MDA-MB-231 cells was determined by 5-ethynyl-2-deoxyuridine (Edu, Invitrogen, USA) incorporation into the cells (Alexa Fluor 488 Imaging Kit, Invitrogen, China) as described previously (22).
Approximately 1×103 cells in total were placed on 96-well plates and cultured for 12 h. Then, we added the medium with CCK-8 solution (Cell Counting Kit-8, Beyotime, China) to each well at the same time. At 450 and 630 nm, we detected the OD values. All experiments were performed in triplicate.
Invasion assay
Approximately 5×104 cells per well were plated into the top Matrigel invasion chambers (Corning, USA) in serum-free medium, while the bottom chambers were filled with serum-containing medium. The cells were allowed to penetrate through the Matrigel and 8 µm pores toward medium containing 15% serum for 24 h. Cells that invaded through the membrane were fixed with 3.7% paraformaldehyde, stained with hexamethyl pararosaniline (Beyotime, China) solution and photographed by an Axio ZoomV16 Zeiss microscope (Jena, Germany).
Migration assay
Culture inserts (Ibidi, Germany) were placed in 35 mm dishes to form standardized wounds. Cells were plated into each well (5×104 cells/well) of culture inserts and incubated for 24 h. The culture inserts were then gently removed. The cells were incubated with culture medium and photographed at the indicated time points. Cell migration capacity was evaluated by measuring the wound healing distance with ImageJ software.
Western blotting
Whole-cell protein lysates were generated using total lysis buffer; nuclear and cytoplasmic protein lysates were generated using buffer A and buffer C as previously described (23). Both protein isolation reagents were supplemented with complete protease inhibitor cocktail (Roche Diagnostics, USA). Lysates were separated on a 15% or 10% acrylamide gel and immobilized on PVDF membranes (Millipore, USA).
The membranes were incubated with primary antibodies [anti-ECSIT (1:1000, Abcam, USA), anti-p53 (1:1,000, Cell Signaling Technology, USA), anti-cleaved capase-3 (1:1,000, Abcam, USA), anti-H3 (1:1,000, Cell Signaling Technology, USA) and anti-GAPDH (1:1,000, Proteintech, USA)] followed by incubation with peroxidase-conjugated secondary antibodies. The signals were detected with an ECL system (Thermo Fisher, USA). The bands were quantified using ImagingJ software.
Cell death (propidium iodide/Hoechst 33342 staining and cleaved caspase-3 staining)
Cells (1×105 cells/well) were seeded into 6-well culture dishes with coverslips covered for 24 h before incubation with propidium iodide (PI, Target Mol, China) solution for 10 min at 37 ℃ followed by 1× PBS washes. Then, the cells were stained with Hoechst 33342 for 10 min at 37 ℃. Fluorescence images were visualized with an Olympus fluorescence microscope and slides were mounted by ImagingJ software.
To examine cell death in the tumor, cryostat sections (4 µm) were cut from tumors and immunolabeled with an antibody against cleaved caspase-3 (Cell Signaling Technology, USA), followed by incubation with related secondary antibodies. Nuclei were counterstained with DAPI. Sections were viewed with a fluorescence microscope. A total of five microscopic fields were photographed and parameters were counted by a blinded investigator.
Xenograft tumor growth in nude mice
Experiments were performed under a project license (No. USTCACUC1801054) granted by the Institutional Animal Welfare Guidelines of University of Science and Technology of China, in compliance with the Animal Care and Use Committee of the University of Science and Technology of China guidelines and Chinese legislation for the care and use of animals. The tumorigenicity assay was performed by subcutaneous injection of 1×106 MDA-MB-231 cells in 80 µL of phosphate-buffered saline and 20 µL of Matrigel matrix (BD Biosciences) into BALB/c nude female mice (4–6 weeks; Nanjing University Laboratory Animal Center, Nanjing, China). The long radius a and short radius b of the tumor were measured with a Vernier caliper every three days, and the tumor volumes were calculated using the following formula: V = 0.52×a×b2.
Immunohistochemistry (IHC) and histological analysis
IHC was performed as previously described (24). Sections were incubated with primary antibody p65 (1:800, Cell Signaling Technology, USA) overnight at 4 ℃. Two experienced pathologists scored p65 IHC staining independently according to the intensity and percentage of p65-positive cells.
Hematoxylin and eosin (H&E)-stained slides were made from xenograft tumors and scanned at ×200 and ×400 magnification.
Statistical analysis
All results are presented as the mean ± SEM. The GraphPad Prism 5.0 (GraphPad, San Diego, CA, USA) software was used to analyze data. Comparisons between groups were performed using either two-tailed student t-test or analysis of continuous variables by one-way analysis of variance (ANOVA), and Tukey’s procedure for multiple range tests was performed. P<0.05 was considered to be significant.
Results
ECSIT regulates human breast cancer cell proliferation, migration, and invasion in vitro
ECSIT acts as an intermediator in the Toll like receptor signaling pathway by interacting with TRAF6 and thus activates NF-κB. Previous investigations have shown that NF-κB can potentially regulate tumor progression, and inhibiting NF-κB activation by NKILA reduces tumor metastasis (17). To study the function of ECSIT in human breast cancer, we overexpressed ECSIT by transfecting a Flag-ECSIT plasmid and established ECSIT-knockdown cell lines using a lentiviral vector carrying short hairpin RNA (shRNA) in MDA-MB-231 cell lines.
Then, the level of ECSIT expression was validated by Western blotting after transfection with Flag-ECSIT and shECSIT in cells (data not shown). Our data showed that overexpression of ECSIT significantly promoted the cell proliferation. In contrast, a significant decrease in cell proliferation rate was observed after knockdown of ECSIT (Figure 1A,1B). Transfecting of Flag-ECSIT accelerated cell migration and invasion meanwhile, in shECSIT cells, the wound closure and invasion speed were remarkably reduced compared with those of the shRandom group (Figure 1C,1D). Collectively, these data indicate that ECSIT could promote human breast cancer development.
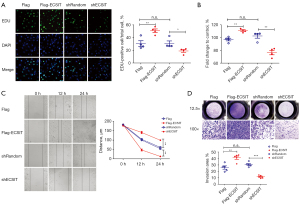
ECSIT regulates cell death in human breast cancer cells via NF-κB and p53
Next, we aimed to confirm whether ECSIT could regulate cell death in MDA-MB-231 cells. Disruption of internal and external membranes is related to cell death. Therefore, we measured cell membrane integrity by PI/Hoechst 33342 staining. Maximum PI uptake and nuclei with Hoechst 33342 binding indicate cell death (25). The data revealed that the percentage of dead cells in the shECSIT group was increased (Figure 2A). Meanwhile, we examined the protein expression level of the key mediator cleaved caspase-3 by Western blot analysis. Knockdown of ECSIT in MDA-MB-231 cells significantly increased the level of cleaved caspase-3 (Figure 2B), suggesting that ECSIT inhibits cell death in breast cancer.
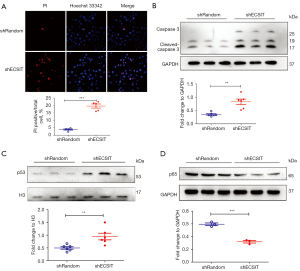
p53 is a nuclear protein that plays an important role in DNA replication, transcription and cell cycle control. Usually, p53 is at a low level in tissues and cells and hard to detect by immunochemical methods (26). Low expression of nuclear p53 in shRandom MDA-MB-231 cells was observed as previously described (20,26). The Western blot analysis showed that the protein expression level of p53 in nuclei was increased by 189.5% in the shECSIT group compared with the shRandom group (Figure 2C).
Both p53 and NF-κB are transcription factors and serve as major cellular hubs. NF-κB is an important transcription factor regulating the inflammatory response (27). Moreover, the cells affected by p53 and NF-κB usually navigate toward opposite fates. The expression level of p65 protein was reduced in the shECSIT group (Figure 2D), suggesting that the nuclear accumulation of p53 is associated with the decreased expression of p65 in MDA-MB-231 cells lacking ECSIT. Therefore, p53 and p65 expression was regulated in response to silencing ECSIT, indicating that the two proteins are involved in ECSIT protein expression in human breast cancer cells.
Silencing ECSIT in breast cancer cells decelerates tumorigenesis
To address whether downexpression of ECSIT decelerated tumorigenesis of human breast cancer, we established a BALB/c nude mouse xenograft model with MDA-MB-231 cells. We find that tumor growth was significantly inhibited in the shECSIT group, as measured by the final xenograft tumor volume and weight (Figure 3A,3B). H&E staining showed that the tumor cell density was decreased and less stained in the shECSIT group (Figure 3C).
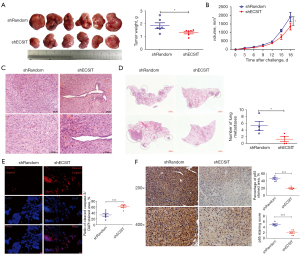
Recently, Quinn reported that NFKB1A, an inhibitor of NF-κB, was a negative metastasis-associated candidate that plays a role in attenuating metastatic potential (28). Therefore, we investigated whether ECSIT influences metastases in vivo. H&E staining analysis of MDA-MB-231 metastasis-bearing lungs showed a significant trend toward a reduced number of lung metastases in the shECSIT group compared with the shRandom group (Figure 3D). Metastases were detectable in 50% of the shECSIT group compared with 100% of the control. These results further supported that inhibiting ECSIT in human breast cancer might reduce the disease progression by decreasing cell proliferation, migration, and invasion and increasing cell death.
Silencing ECSIT in breast cancer cells increased cell death and downregulated p65 expression
We next investigated the effect of ECSIT on cell death in a mouse xenograft model. The cleaved caspase-3 positive areas were significantly increased in the shECSIT group according to the immunological fluorescent staining, and this result was consistent with the in vitro cell data (Figure 3E). Silencing ECSIT also significantly reduced the expression level of p65 in the tumors of MDA-MB-231-bearing mice. There were fewer p65-positive cells in the tumor, as shown by IHC staining, than in the shRandom group (Figure 3F). Together, these data further validated the essential role of ECSIT in cell death and the regulation of p65 in vivo.
The role of ECSIT in cell death was dependent on p53
To examine whether ECSIT induced cell death in MDA-MB-231 cells through a p53 dependent mechanism, we used small interfering RNA (sip53) to knockdown the expression of p53 before the cells were examined by PI/Hoechst 33342 staining. Deletion of p53 abolished the increase in cell death induced by shECSIT (Figure 4A). To confirm the contribution of p53 to ECSIT-induced cell death, we used Western blotting to detect the cell apoptosis signaling pathway in vitro. In the siNC groups, the level of cleaved caspase-3 was increased after knockdown of ECSIT in MDA-MB-231 cells compared with shRandom cells. However, the role of ECSIT was abolished in cells that were treated with sip53. These data suggest that p53 is required for ECSIT-induced cell death (Figure 4B).
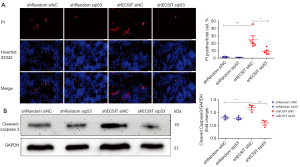
Discussion
Cell death is a process of fundamental importance to human health and has been observed in cancers for a long time. Problems in cell death and mutation that promote aberrant cell proliferation will cause rapid growth of nascent malignant clones (29). There are different types of cell death because cancer can deny death to keep the immune system at bay (30). It is important to understand cell death signaling to improve the efficiency of cancer treatment.
In this study, our data represent heretofore the first clear demonstration that ECSIT plays a significant role in the progression and metastasis of human breast cancer. Regulation of ECSIT in MDA-MB-231 cells affects cell proliferation, viability, migration, invasion, and death. Knockdown of ECSIT in MDA-MB-231 cells also increased p53 expression and decreased the expression of p65 in nuclei. Inhibition of p53 by siRNA prevented ECSIT-induced cell death, indicating that ECSIT might be involved in breast cancer progression through a p53-dependent pathway. Furthermore, in a breast cancer xenograft mouse model, knockdown of ECSIT induced cell death and depressed tumorigenicity and metastasis.
TNBC is a kind of breast cancer that does not have estrogen or progesterone receptors and HER2 overexpression and is commonly found in breast cancer. Additionally, TNBC patients have a short progression-free survival due to their higher rates of metastasis (31). Initially, we chose the MCF-7, 4T-1 and MDA-MB-231 cell lines to detect the effect of ECSIT in breast cancer. We found that ECSIT barely regulated cell proliferation, invasion and migration in MCF-7 cells and had no function in tumor progression in 4T-1-bearing mice (Figures S1,S2). Importantly, we thought the MDA-MB-231 cell line was more representative, and it is a highly aggressive, invasive TNBC cell line.
ECSIT is an intermediate in the TLR/IL-1R signal transduction pathway that is required for NF-κB activation and interacts with TRAF 6 via its amino acids 137-435 (13). Endogenous ECSIT interacts with p65/p50 NF-κB proteins in the nucleus to form a molecular complex after TLR4 stimulation. In particular, knockdown of ECSIT in THP-1 cells inhibits DNA-binding activities of p65 and p50 (16). These results are consistent with our result where decreased expression of p65 occurs in shECSIT MDA-MB-231 cells and tumors of MDA-MB-231-bearing mice in the shECSIT group. We thought that ECSIT is essential for p65 expression in tumorigenesis, and the mechanism by which ECSIT regulates p65 translation will be the focus of in our further research.
In many tumors, NF-κB is constitutively active and plays a key role in cancer development (32), and downregulating the NF-κB pathway has been shown to suppress tumors (33,34). Our data indicate that knockdown of ECSIT in MDA-MB-231 cells has an effect similar to that of an NF-κB suppressor by inhibitions cancer cell proliferation, migration and invasion and promotes cell death. Furthermore, in numerous physiological studies, cross talk between the p53 and NF-κB pathways has been studied. Loss of p53 function is associated with most human cancers (21) and prevents cells from undergoing apoptosis (35). Yin et al. revealed that miR-506 downregulates p65 and stimulates p53 expression in lung cancer cells (36). In our study, we found an increased level of p53 and a decrease in p65 in the shECSIT group, which implies that ECSIT induces cell death and promotes tumorigenesis by inhibiting p53 and activating p65; the clear mechanism will be examined in more detail in our next study. Taken together, our data provide a novel target for human breast cancer therapy, and the development of novel ECSIT inhibitors is important for the management of TNBC.
Acknowledgments
This work was supported by Prof. Ge Shan, who provided the shECSIT plasmid.
Funding: We gratefully acknowledge the financial support from
Footnote
Reporting Checklist: The authors have completed the MDAR reporting checklist. Available at https://tcr.amegroups.com/article/view/10.21037/tcr-21-2234/rc
Data Sharing Statement: Available at https://tcr.amegroups.com/article/view/10.21037/tcr-21-2234/dss
Conflicts of Interest: All authors have completed the ICMJE uniform disclosure form (available at https://tcr.amegroups.com/article/view/10.21037/tcr-21-2234/coif). YH reports receiving funding support from the National Natural Science Foundation of China (No. 81700232). DL reports receiving funding support from the National Natural Science Foundation of China (No. 32090041, 21778050, 91749125), Major Research Plan of the National Natural Science Foundation of China (No. 2016YFA0101200), New Medical Science Joint Fund of USTC (No. WK2070000123) and Training Program of the Major Foundation of USTC (No. WK3520000007). The other authors have no conflicts of interest to declare.
Ethical Statement: The authors are accountable for all aspects of the work in ensuring that questions related to the accuracy or integrity of any part of the work are appropriately investigated and resolved. The study was conducted in accordance with the Declaration of Helsinki (as revised in 2013). Experiments were performed under a project license (No. USTCACUC1801054) granted by the Institutional Animal Welfare Guidelines of University of Science and Technology of China, in compliance with the Animal Care and Use Committee of the University of Science and Technology of China guidelines and Chinese legislation for the care and use of animals.
Open Access Statement: This is an Open Access article distributed in accordance with the Creative Commons Attribution-NonCommercial-NoDerivs 4.0 International License (CC BY-NC-ND 4.0), which permits the non-commercial replication and distribution of the article with the strict proviso that no changes or edits are made and the original work is properly cited (including links to both the formal publication through the relevant DOI and the license). See: https://creativecommons.org/licenses/by-nc-nd/4.0/.
References
- DeSantis CE, Ma J, Gaudet MM, et al. Breast cancer statistics, 2019. CA Cancer J Clin 2019;69:438-51. [Crossref] [PubMed]
- Sung H, Ferlay J, Siegel RL, et al. Global Cancer Statistics 2020: GLOBOCAN Estimates of Incidence and Mortality Worldwide for 36 Cancers in 185 Countries. CA Cancer J Clin 2021;71:209-49. [Crossref] [PubMed]
- Li G, Hu J, Hu G. Biomarker Studies in Early Detection and Prognosis of Breast Cancer. Adv Exp Med Biol 2017;1026:27-39. [Crossref] [PubMed]
- Early Breast Cancer Trialists' Collaborative Group (EBCTCG). Relevance of breast cancer hormone receptors and other factors to the efficacy of adjuvant tamoxifen: patient-level meta-analysis of randomised trials. Lancet 2011;378:771-84. [Crossref] [PubMed]
- Swain SM, Baselga J, Kim SB, et al. Pertuzumab, trastuzumab, and docetaxel in HER2-positive metastatic breast cancer. N Engl J Med 2015;372:724-34. [Crossref] [PubMed]
- Mittendorf EA, Philips AV, Meric-Bernstam F, et al. PD-L1 expression in triple-negative breast cancer. Cancer Immunol Res 2014;2:361-70. [Crossref] [PubMed]
- Bardia A, Mayer IA, Diamond JR, et al. Efficacy and Safety of Anti-Trop-2 Antibody Drug Conjugate Sacituzumab Govitecan (IMMU-132) in Heavily Pretreated Patients With Metastatic Triple-Negative Breast Cancer. J Clin Oncol 2017;35:2141-8. [Crossref] [PubMed]
- Gyrd-Hansen M, Meier P. IAPs: from caspase inhibitors to modulators of NF-kappaB, inflammation and cancer. Nat Rev Cancer 2010;10:561-74. [Crossref] [PubMed]
- Nathan C, Ding A. Nonresolving inflammation. Cell 2010;140:871-82. [Crossref] [PubMed]
- Grivennikov SI, Greten FR, Karin M. Immunity, inflammation, and cancer. Cell 2010;140:883-99. [Crossref] [PubMed]
- Li S, Lv M, Qiu S, et al. NF-κB p65 promotes ovarian cancer cell proliferation and migration via regulating mortalin. J Cell Mol Med 2019;23:4338-48. [Crossref] [PubMed]
- Zhang YC, Huo FC, Wei LL, et al. PAK5-mediated phosphorylation and nuclear translocation of NF-κB-p65 promotes breast cancer cell proliferation in vitro and in vivo. J Exp Clin Cancer Res 2017;36:146. [Crossref] [PubMed]
- Kopp E, Medzhitov R, Carothers J, et al. ECSIT is an evolutionarily conserved intermediate in the Toll/IL-1 signal transduction pathway. Genes Dev 1999;13:2059-71. [Crossref] [PubMed]
- Moustakas A, Heldin CH. Ecsit-ement on the crossroads of Toll and BMP signal transduction. Genes Dev 2003;17:2855-9. [Crossref] [PubMed]
- West AP, Brodsky IE, Rahner C, et al. TLR signalling augments macrophage bactericidal activity through mitochondrial ROS. Nature 2011;472:476-80. [Crossref] [PubMed]
- Mi Wi S, Park J, Shim JH, et al. Ubiquitination of ECSIT is crucial for the activation of p65/p50 NF-κBs in Toll-like receptor 4 signaling. Mol Biol Cell 2015;26:151-60. [Crossref] [PubMed]
- Liu B, Sun L, Liu Q, et al. A cytoplasmic NF-κB interacting long noncoding RNA blocks IκB phosphorylation and suppresses breast cancer metastasis. Cancer Cell 2015;27:370-81. [Crossref] [PubMed]
- Barbie TU, Alexe G, Aref AR, et al. Targeting an IKBKE cytokine network impairs triple-negative breast cancer growth. J Clin Invest 2014;124:5411-23. [Crossref] [PubMed]
- Yamaguchi N, Ito T, Azuma S, et al. Constitutive activation of nuclear factor-kappaB is preferentially involved in the proliferation of basal-like subtype breast cancer cell lines. Cancer Sci 2009;100:1668-74. [Crossref] [PubMed]
- Vousden KH, Lu X. Live or let die: the cell's response to p53. Nat Rev Cancer 2002;2:594-604. [Crossref] [PubMed]
- Vogelstein B, Lane D, Levine AJ. Surfing the p53 network. Nature 2000;408:307-10. [Crossref] [PubMed]
- Song J, Zhu Y, Li J, et al. Pellino1-mediated TGF-β1 synthesis contributes to mechanical stress induced cardiac fibroblast activation. J Mol Cell Cardiol 2015;79:145-56. [Crossref] [PubMed]
- Hu Y, Zhang M, Shen X, et al. TIR/BB-loop mimetic AS-1 attenuates cardiac ischemia/reperfusion injury via a caveolae and caveolin-3-dependent mechanism. Sci Rep 2017;7:44638. [Crossref] [PubMed]
- Wu W, Hu Y, Li J, et al. Silencing of Pellino1 improves post-infarct cardiac dysfunction and attenuates left ventricular remodelling in mice. Cardiovasc Res 2014;102:46-55. [Crossref] [PubMed]
- George J, Gondi CS, Dinh DH, et al. Restoration of tissue factor pathway inhibitor-2 in a human glioblastoma cell line triggers caspase-mediated pathway and apoptosis. Clin Cancer Res 2007;13:3507-17. [Crossref] [PubMed]
- Harris AL. p53 expression in human breast cancer. Adv Cancer Res 1992;59:69-88. [Crossref] [PubMed]
- Belvin MP, Anderson KV. A conserved signaling pathway: the Drosophila toll-dorsal pathway. Annu Rev Cell Dev Biol 1996;12:393-416. [Crossref] [PubMed]
- Quinn JJ, Jones MG, Okimoto RA, et al. Single-cell lineages reveal the rates, routes, and drivers of metastasis in cancer xenografts. Science 2021;371:eabc1944.
- Strasser A, Vaux DL. Cell Death in the Origin and Treatment of Cancer. Mol Cell 2020;78:1045-54. [Crossref] [PubMed]
- Legrand AJ, Konstantinou M, Goode EF, et al. The Diversification of Cell Death and Immunity: Memento Mori. Mol Cell 2019;76:232-42. [Crossref] [PubMed]
- Lee A, Djamgoz MBA. Triple negative breast cancer: Emerging therapeutic modalities and novel combination therapies. Cancer Treat Rev 2018;62:110-22. [Crossref] [PubMed]
- DiDonato JA, Mercurio F, Karin M. NF-κB and the link between inflammation and cancer. Immunol Rev 2012;246:379-400. [Crossref] [PubMed]
- Boldin MP, Baltimore D. MicroRNAs, new effectors and regulators of NF-κB. Immunol Rev 2012;246:205-20. [Crossref] [PubMed]
- Ruland J. Return to homeostasis: downregulation of NF-κB responses. Nat Immunol 2011;12:709-14. [Crossref] [PubMed]
- Janic A, Valente LJ, Wakefield MJ, et al. DNA repair processes are critical mediators of p53-dependent tumor suppression. Nat Med 2018;24:947-53. [Crossref] [PubMed]
- Yin M, Ren X, Zhang X, et al. Selective killing of lung cancer cells by miRNA-506 molecule through inhibiting NF-κB p65 to evoke reactive oxygen species generation and p53 activation. Oncogene 2015;34:691-703. [Crossref] [PubMed]