Natural product piperlongumine inhibits proliferation of oral squamous carcinoma cells by inducing ferroptosis and inhibiting intracellular antioxidant capacity
Introduction
Oral squamous cell carcinoma (OSCC) is the most common head and neck tumor, accounting for more than four fifths of oral malignant tumors. The global incidence is widespread, with 450,000 cases and 230,000 deaths reported every year, with poor prognosis (1,2). Surgery has always been the first-line treatment in OSCC, whether early or late. However, due to limited medical resources, some patients are still unable to receive surgical treatment in time (3). The non-surgical treatment of OSCC mainly includes radiotherapy, chemoradiotherapy and immunotherapy. Although significant progress has been made in the treatment of OSCC, most are still in local advanced stage with poor prognosis, and the average 5-year survival rate is less than 50% to 60% (4). One of the main reasons for this result is that OSCC cells are progressively resistant to the currently available chemotherapeutic drugs (5). Therefore, there is an urgent need for new treatment methods.
The term ferroptosis was first coined by Brent R. Stockwell in 2012 to describe an iron dependent, non-apoptotic cell death (6). The typical characteristics of ferroptosis include the dependence of active Fe2+ and the accumulation of a large number of phospholipid hydroperoxides in membrane phospholipids (PL-PUFAs) (7), which is of great significance for the treatment of tumors resistant to existing chemotherapeutic drugs. In the process of ferroptosis, due to the insufficient uptake of glutamate, the content of intercellular glutathione (GSH) decreases, resulting in Lipid peroxidation (LPO). At present, it has been confirmed that tumor cells have more Fe2+ than normal cells, with a greater demand for glutamate (8). Therefore, guiding the existing cancer cells to ferroptosis is a new treatment strategy with unlimited potential.
As a natural alkaloid extracted from pepper, piperlongumine (PL) has found a variety of biological activities, including antiplatelet, antidepressant, neuroprotection, anti-atherosclerosis, antibacterial and so on (9). In addition, it has been found that PL has significant anticancer effect and has been identified as a powerful cytotoxic element with high selectivity to cancer cells (10). PL is cytotoxic to many cancer cell lines, such as colon cancer, lung cancer, breast cancer, pancreatic cancer, and renal cell carcinoma. Furthermore, antitumor activity was found in a variety of animal models (9). Interestingly, PL has very little toxicity to human normal cells as a natural herbal medicine (11). There are many ways for PL to kill tumor cells, including increasing the level of intracellular reactive oxygen species [ROS, a group of molecules derived from molecular oxygen, mainly including hydrogen peroxide (H2O2), singlet oxygen (1O2), superoxide anion (O2•–) and hydroxyl radicals (•OH)], cell cycle arrest and regulating autophagy. Recently, PL has been found to induce ferroptosis (12). However, the effect and mechanism of PL on OSCC cells have not been reported.
The energy demand of tumor cells is significantly higher than that of normal cells. Based on this situation, anti-metabolic therapy has become the mainstream of tumor treatment. Among them, glutamine metabolism has received particular attention in this context (13). In addition, the root cause of LPO in ferroptosis is the insufficient content of GSH, which comes from the lack of raw materials for its synthesis - glutamate, cysteine and glycine. The vast majority of intracellular glutamate comes from glutamine decomposition, in which Glutaminase (GLS) is the rate limiting enzyme. Therefore, many kinds of GLS inhibitors have been developed to treat malignant tumors (14). Since 2014, CB-839 (telaglenstat), as a new GLS1 inhibitor, has been proved to have anticancer effect on melanoma, multiple myeloma, lymphoma and renal cell carcinoma, and is the only glutaminase inhibitor that has entered clinical trials (15). High metabolic level makes tumor cells need more GSH, while CB-839 can reduce glutamate content by inhibiting glutamine decomposition, therefore, the combination of PL with CB-839 may enhance the antitumor effect of PL.
In this study, we tested the possibility of PL to induce ferroptosis on OSCC. We also evaluated the synergistic effect of CB-839 on PL induced ferroptotic death of OSCC cells. Our study suggested that PL alone could induce ferroptosis of OSCC cells and the combination of PL with CB-839 may further enhance the ferroptosis. Our study provide a new strategy for OSCC treatment. We present the following article in accordance with the MDAR reporting checklist (available at https://tcr.amegroups.com/article/view/10.21037/tcr-22-1494/rc).
Methods
Cell lines and reagents
Oral squamous cell carcinoma cell lines (HSC-3 and H400) were obtained from the Japanese Collection of Research Bioresources (JCRB) Cell Bank. The cells were routinely cultured in DMEM supplemented with 10% fetal bovine serum (Invitrogen Life Technologies) and 1% antibiotics at 37 ℃ in 5% CO2 incubator. PL was purchased from MedChemExpress and was dissolved in dimethyl sulfoxide (DMSO). The primary antibodies anti-GPX4, anti-FTH1, anti-xCT/SLC7A11, anti-DMT1/SLC11A2 were obtained from Cell Signaling Technology (1:1,000). GAPDH were purchased from Zenbio (1:1,000). Goat anti-mouse/rabbit secondary antibody was purchased from Cell Signaling Technology. Ferrostatin-1 was purchased from Topscience. N-Acetylcysteine (NAC), DCFH-DA and CB-839 were purchased from MedChemExpress.
Cell counting kit-8 (CCK-8) assay
Cells were reseeded into 96-well plates at a density of 5×103 cells per well. The cells were then treated with various concentrations of PL (0, 1, 5, 10, 15, 20, 30, 40, 50 µM), Fer-1, NAC for 24 and 48 hr. Then, the medium was replaced with CCK-8 solution (Dojindo Molecular Technologies, DJDB4000X) and incubated at 37 ℃ in the dark for 2–4 hr. The absorbance was measured by Varioskan Flash (Thermo Scientific) at a wavelength of 450 nM.
Flow cytometric assay
Intracellular ROS assay: both HSC-3 and H400 cells were treated in the absence or presence of PL, Fer-1, NAC and incubated for 24 hr, harvested and resuspended in PBS. Cells were incubated with 10 µM DCFH-DA (MedChemExpress) for 30 min in the dark. Finally, the fluorescence intensity of the 2,7-dichlorofluorescein dye was measured using flow cytometry (Beckman FC500).
Flow cytometry was also used to measure cell apoptosis by using an Annexin V-FITC/PI apoptosis detection kit (Nanjing Keygen Biotech Co. Ltd.) according to the manufacturer’s instructions.
LPO malondialdehyde (MDA) assay
Cells were seeded in petri dishes and incubated overnight at 37 ℃. Cells were treated in the absence or presence of PL, Fer-1, NAC and CB-839 for 24 hr, harvested and resuspended in PBS. The number of cells in each dish was counted with a cell counter. MDA was measured by using a LPO MDA Assay Kit (Beyotime Biotechnology Co. Ltd.) according to the manufacturer’s instructions.
Oxidized glutathione (GSH) assay
Cells were seeded in petri dishes and incubated overnight at 37 ℃. Cells were treated in the absence or presence of PL, Fer-1, NAC and CB-839 for 24 hr, harvested and resuspended in PBS. GSH was measured by using a GSH and GSSG Assay Kit (Beyotime Biotechnology Co. Ltd.) according to the manufacturer’s instructions.
Western blot analysis
HSC-3 and H400 cells were stimulated with PL for 6, 12 and 24 hr. The proteins were gathered in each group. Western blot analysis was performed as elsewhere (16). The primary antibodies were described above. The results were visualized by using an enhanced chemiluminescence Western blotting detection reagent (Millipore). Plots were quantified using ImageJ software. Protein band intensities were normalized to GAPDH, and data were expressed as the percent relative to controls.
Statistical analysis
All experiments were carried out independently at least three times. The results are calculated as the means ± the standard deviation (SD) using GraphPad Prism software (version 5.3; GraphPad Software, Inc., La Jolla, CA, USA). The differences between the experimental groups and controls were assessed by single-factor analysis of variance (ANOVA) and Student’s t-test. A P value less than 0.05 (P<0.05) was regarded as a statistically significant result.
Results
PL inhibits proliferation in OSCC cells and induces apoptosis
In order to evaluate the effect of PL on the proliferation of OSCC cells, HSC-3 and H400 were treated with different concentrations of PL for 24 and 48 h respectively, and the cell viability was displayed by CCK-8. As shown in Figure 1A, after H400 was treated with 5 and 10 µM PL for 24 hours, the cell viability increased slightly for an unknown reason. However, the viability of the two cells decreased significantly with the increase of PL treating concentration, which proved the dose-dependent cytotoxicity of PL to OSCC cells. At the same time, under the same concentration of PL, the cell viability of PL-treated cells for 48 h was lower than that for 24 h, the IC50 values of PL for the HSC-3 cells were 14.57 µM (24 hr), 4.02 µM (48 hr), respectively. For the H400 cells, the IC50 values were 28.34 µM (24 hr), 14.71 µM (48 hr), correspondingly. Therefore, the time-dependent cytotoxicity of PL to OSCC cells was proved. It is explained here that the PL concentration of subsequent experiments is based on the IC50 values of PL for the HSC-3 cells was 15 µM, for the H400 cells was 30 µM.
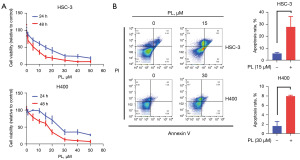
To further determine the effectiveness of PL in OSCC cells, the apoptosis rate was tested 24 hr after cells were treated with PL. PL treatment for 24 hr dramatically induced both early and late apoptosis in OSCC cells (Figure 1B).
PL induced intracellular oxidative stress
Many studies have shown that PL can promote the accumulation of ROS in some tumor cells, such as lung cancer (17), hepatocellular carcinoma (18). To determine the effect of PL on OSCC cells, we hypothesized that PL could also induce oxidative stress in OSCC cells. In order to test the hypothesis, the content of ROS and MDA were measured by flow cytometry after cells were treated by PL. As shown in Figure 2, the content of ROS and MDA in PL-treated HSC-3 and H400 cells both increased significantly. MDA is one of the end products of intracellular oxidative stress. After ROS is produced, it can act on the PL-PUFAs in the cell membrane and oxidize it to MDA (7). Therefore, the current results suggest that PL may inhibit the proliferation of OSCC cells by inducing oxidative stress.
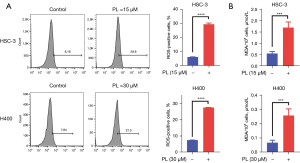
PL affected the expression of ferroptosis related proteins in OSCC cells
An existing study has shown that PL can induce ferroptosis in some cells (19), therefore, we hypothesized that PL may inhibit the proliferation of OSCC cells by being an activator of ferroptosis. To test this hypothesis, we measured the changes of some ferroptosis key proteins after PL treatment. DMT1, as a component of solution carrier family 11 member 2, helps Fe2+ release into the cytoplasm and increases the content of labile iron pool (LIP) (20). The iron-storage protein ferritin includes ferritin light chain (FTL) and ferritin heavy chain 1 (FTH1), which can be degraded by lysosomes to increase free iron levels. The amino acid antiporter SLC7A11/xCT/system xc− can take in cystine, which is used to synthesize GSH. GPX4 functions as a phospholipid hydroperoxidase to reduce phospholipid hydroperoxide production (ROOH) to the corresponding phospholipid alcohol (ROH). When SLC7A11 and GPX4 are inhibited, redox homeostasis is broken, resulting in ferroptosis. For this purpose, three time points, 6, 12 and 24 hours, were set to measure the protein content respectively. As shown in Figure 3, after HSC-3 and H400 cells were treated with PL for 6, 12 and 24 hours, the protein content in the cells changed accordingly. Although some proteins do not change significantly, there were statistically significant changes, which is compared with the control group, the expression of DMT1 in OSCC cells treated with PL increased, and the expression of FTH1, SLC7A11 and GPX4 decreased. This is consistent with the characteristics of ferroptosis. Fe2+ is released after FTH1 is degraded by lysosomes, and is transported into the cytoplasm by DMT1, increasing the LIP. At the same time, the contents of SLC7A11 and GPX4 are reduced, resulting in the decline of cell antioxidant capacity. This may be the main cause of PL-induced OSCC cell death, which is consistent with the ferroptosis mechanism.
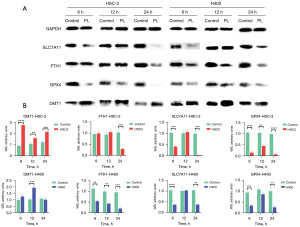
The effect of PL on OSCC cells can be reversed by iron scavengers and antioxidants
If PL can induce ferroptosis in OSCC cells, we hypothesized that iron scavengers and antioxidants could reverse this phenomenon. To further prove the hypothesis, cells were treated with PL combined with Fer-1 or NAC, and observed their effects on cell proliferation, ROS and MDA. As shown in Figure 4A, compared with the cells treated with PL alone, the combined use of Fer-1 or NAC can weaken the inhibitory effect of PL on the proliferation of OSCC cells, and this effect will not weaken with the increase of PL concentration. For H400 cells, the combined treatment with PL and Fer-1 showed the effect of promoting cell proliferation, which probably because H400 cells are more sensitive to Fer-1, which completely offset the effect of PL. Figure 4B shows that ROS decreased significantly after the cells were treated with PL and NAC. However, after the combined treatment of PL and Fer-1, ROS did not decrease significantly or even increased. This may be because the production of ROS in the ferroptosis pathway is upstream of LPO (21). Fer-1 only inhibits LPO, but cannot affect the formation of ROS. Figure 4C shows the MDA content after the combined treatment of Fer-1 and NAC. The results show that both Fer-1 and NAC can reduce the MDA level. These evidences show that PL induces intracellular oxidative stress which can be reversed by iron scavengers and antioxidants, which proves that PL can induce ferroptosis in OSCC cells.
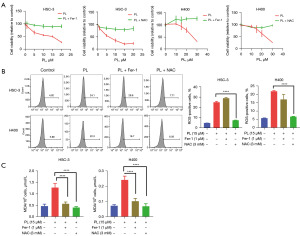
CB-839 can enhance the ferroptosis-inducing effect of PL on OSCC cells
It has been confirmed that PL can induce ferroptosis in OSCC cells, in which PL can inhibit GSH synthesis and GPX4 activity and participate in intracellular glutamine metabolism. CB-839 is a glutaminase inhibitor, therefore, we hypothesized that CB-839 could enhance the effect of PL. Cells were firstly treated with a fixed concentration of PL combined with different concentrations of CB-839, and the most obvious effect of CB-839 concentration was shown in Figure 5A. Compared with the group treated with PL only, 15 µM CB-839 has the highest inhibitory effect on HSC-3, 1 µM CB-839 had the highest inhibitory effect on H400, so we chose these two concentrations of CB-839 for follow-up experiments. After fixing the concentration of CB-839, cells were treated with different concentrations of PL combined with CB-839, as shown in Figure 5B. Compared with the group treated with PL only, treatment with PL and CB-839 synergistically reduced the viability of the cells to more than 10%. However, with the increase of PL concentration, the effect of CB-839 did not change significantly, which means that CB-839 may only increase the proliferation inhibition effect of PL to a certain extent. In order to observe the effect of CB-839 on PL, we measured the MDA content, as shown in Figure 5C. After combined treatment with CB-839, MDA increased significantly compared with the group treated with PL or CB-839 only, indicating that CB-839 can improve the level of intracellular oxidative stress. Further, we measured the GSH content and found that compared with the control group (Figure 5D), PL can significantly reduce intracellular GSH. After combined with CB-839, the level of intracellular GSH further decreased, which not only shows that PL can inhibit the synthesis of intracellular GSH to induce ferroptosis, but also proves that CB-839 can enhance the effect of PL by affecting glutamine metabolism.
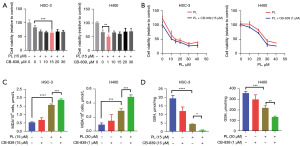
Discussion
At present, OSCC is still one of the main cancers perplexing human beings, with a poor prognosis of advanced patients. In addition, the emergence of drug-resistant strains urgently requires better drug treatment. Regulated cell death (RCD), such as apoptosis, is a recognized hindrance to tumorigenesis. Ferroptosis is a recently identified form of RCD driven by iron-dependent LPO, which is distinct from other RCDs, such as apoptosis, autophagy and necroptosis, in morphology and mechanisms. PL-PUFAs in cellular membranes are susceptible for peroxidation under iron- and ROS-rich conditions. Toxic accumulation of such lipid peroxides in cellular membranes ends up damaging the integrity of the membrane, leading to ferroptosis (22). In recent years, this new form of cell death has been increasingly used in cancer therapy research. As a natural pepper extract, PL has little toxicity to human normal cells, but can selectively induce the death of a variety of cancer cells, making it a good candidate for cancer treatment. It has been shown to promote cell death by activating several mechanisms, including apoptosis, autophagy and necrosis, affecting the PI3K/AKT/mTOR, p38/JNK, MEK/ERK and NF-κB pathways (23). However, the influence of PL on survival of OSCC cells has not been investigated. Our study showed that PL could induce OSCC cell death by increasing ROS, inducing oxidative stress and LPO, which was the same as the previous study (24).
The typical characteristics of ferroptosis are iron dependence and LPO, which contain a few key proteins. In iron metabolism, FTH1 and DMT1 provide and transport iron respectively. After FTH1 is degraded in lysosomes, free Fe2+ is produced, and is transported into the cytoplasm through DMT1 for subsequent metabolism (Figure 6). The production of ROS is the basis of lipid metabolism, and the lack of GSH production is the primary cause of ROS generation. When SLC7A11 and GPX4 are inhibited, GSH production is insufficient, and the remaining GSH cannot be catalyzed by GPX4 to ROOH. In this study, we found that PL could reduce the expression of FTH1, SLC7A11 and GPX4 in OSCC cells, while the expression of DMT1 increased, which was consistent with the metabolic process of ferroptosis (25). After treatment with iron scavengers and antioxidants, the inhibitory effect of PL on cell proliferation was weakened, which also showed that PL induced ferroptosis of OSCC cells.
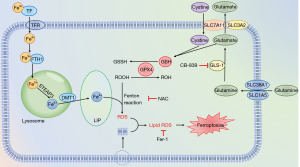
In general, Fe3+ binds to transferrin (TF), entering the cells through transferrin receptor (TFRC), and is reduced to Fe2+ by endosomal six-transmembrane epithelial antigen of prostate 3 (STEAP3). Free Fe2+ is transported into the cytoplasm by DMT1, participating in the formation of LIP, or being stored with FTH1 (Figure 6). Subsequent LPO is provoked by excessive iron either by the iron-mediated Fenton reaction to produce ROS and capture hydrogen atoms from PL-PUFAs, or activating enzymes containing iron (for example, lipoxygenases) (26,27). As a radical-trapping antioxidant (28), Fer-1 is identified from screenings as potent ferroptosis inhibitors, and its activity lies in its capability to slow down the accumulation of lipid hydrogen peroxide (29). However, our results showed that compared with the group treated with PL only (as shown in Figure 4B), the content of ROS in cells treated with PL and Fer-1 did not change significantly (P>0.05), but the content of MDA decreased. This can be interpreted as Fer-1 inhibits LPO, but not mitochondrial ROC formation or lysosomal membrane permeability (30). Specifically, the electron leakage from electron transport chain complexes I and III produces superoxide (O2•−), which is subsequently converted to H2O2. H2O2 react rapidly with oxygen to form PL-PUFA peroxyl radicals (PL-PUFA-OO•) through Fenton reaction, which ultimately form PL-PUFA hydroperoxides (PL-PUFA-OOH) (28). It is reported that Fer-1 may release two protons and two electrons, through the tautomer intermediate compound A, resulting in the formation of a redox-stable compound B (30). This mechanism could form the basis for the anti-LPO activity of Fer-1. N-acetyl cysteine, which is a GSH precursor, protects against various oxidative cell deaths, including ferroptosis. Therefore, after the cells were treated with PL and NAC, both ROS and MDA decreased.
An abundant supply of amino acids is critical to sustain highly proliferative tumor tissues, and reprogramming of amino acid metabolism emerged as a valuable source of biomarkers and therapeutic targets for cancer treatment (13). Most glutamate in cells comes from glutamine decomposition, in which GSL1 acts as a catalyst, and the generated glutamate is subsequently involved in the formation of GSH. Lang et al. found that the expression of GLS1 generally increased in head and neck squamous cell carcinoma, so inhibiting GLS1 may be a more effective treatment. CB-839 selectively inhibits GLS1 but not GLS2 (Figure 6), and as a monotherapy it has only a very limited anticancer effect (31). Therefore, in this experiment, we combined PL with CB-839. Interestingly, compared with the group treated with PL only, the combination of CB-839 can further inhibit the proliferation of OSCC cells, reduce the content of GSH, promote LPO and lead to ferroptosis. This also suggests that PL combined with CB-839 may be a more superior treatment scheme.
At present, there are more and more studies on ferroptosis, and its metabolic process and regulatory factors are more and more clear. In this study, we only measured the key proteins in ferroptosis metabolism, which are DMT1, FTH1, SLC7A11 and GPX4, but there are many other proteins related to ferroptosis that need to be studied. SLC38A1/SLC1A5 complex exists on cell membrane and participates in glutamine uptake. Liu et al. found that the expression of SLC38A1 increased in hepatoma cells, and the upregulation of SLC38A1 expression predicted a worse prognosis for HCC patients (32). Cao et al. found that SLC1A5 expression was elevated in various solid cancers, and inhibition of its expression could induce ferroptosis in breast cancer cells (33). All these researches indicate that SLC38A1/SLC1A5 complex may be one of the new targets for tumor therapy. It has been reported that the inhibition of ROS-independent molecular targets of PL, such as PI3K/Akt/mTOR inhibition (34), can induce the sensitivity of cells to ferroptosis. Mechanistically, AKT up-regulates the expression of mTORC1 (Figure 6), which in turn up-regulates SREBP1 (central regulator of lipid synthesis), promoting lipid repair. Furthermore, PL increases the efficacy of the chemotherapeutics 5-fluorouracil, cisplatin, doxorubicin, paclitaxel, and curcumin, increasing intracellular ROS levels, and enhancing the radiosensitivity of cancer cells (34). However, the real target of PL increasing ROS—a molecule or a protein—has not been explored. What’s more, since PL is highly selective to tumor cells without damaging normal cells, whether there are PL specific receptors on tumor cells is a problem to be studied in the future.
Conclusions
In conclusion, our study demonstrates that PL can induce ferroptosis in OSCC cells. Moreover, the combination of PL and CB-839 enhances the anti-proliferative and ferroptosis-inducing effect of PL. Our results provide a greater understanding of the mechanism by which PL affects OSCC cells and may be of benefit in the development of methods using PL and CB-839 for the treatment of OSCC.
Acknowledgments
Funding: This study was supported by
Footnote
Reporting Checklist: The authors have completed the MDAR reporting checklist. Available at https://tcr.amegroups.com/article/view/10.21037/tcr-22-1494/rc
Data Sharing Statement: Available at https://tcr.amegroups.com/article/view/10.21037/tcr-22-1494/dss
Conflicts of Interest: All authors have completed the ICMJE uniform disclosure form (available at https://tcr.amegroups.com/article/view/10.21037/tcr-22-1494/coif). The authors have no conflicts of interest to declare.
Ethical Statement: The authors are accountable for all aspects of the work in ensuring that questions related to the accuracy or integrity of any part of the work are appropriately investigated and resolved.
Open Access Statement: This is an Open Access article distributed in accordance with the Creative Commons Attribution-NonCommercial-NoDerivs 4.0 International License (CC BY-NC-ND 4.0), which permits the non-commercial replication and distribution of the article with the strict proviso that no changes or edits are made and the original work is properly cited (including links to both the formal publication through the relevant DOI and the license). See: https://creativecommons.org/licenses/by-nc-nd/4.0/.
References
- Wang W, Adeoye J, Thomson P, et al. Statistical profiling of oral cancer and the prediction of outcome. J Oral Pathol Med 2021;50:39-46. [Crossref] [PubMed]
- Siegel RL, Miller KD, Jemal A. Cancer statistics, 2020. CA Cancer J Clin 2020;70:7-30. [Crossref] [PubMed]
- Forner D, Noel CW, Wu V, et al. Nonsurgical management of resectable oral cavity cancer in the wake of COVID-19: A rapid review and meta-analysis. Oral Oncol 2020;109:104849. [Crossref] [PubMed]
- Gangopadhyay A, Bhatt S, Nandy K, et al. Survival Impact of Surgical Resection in Locally Advanced T4b Oral Squamous Cell Carcinoma. Laryngoscope 2021;131:E2266-74. [Crossref] [PubMed]
- Li H, Zhang Y, Xu M, et al. Current trends of targeted therapy for oral squamous cell carcinoma. J Cancer Res Clin Oncol 2022;148:2169-86. [Crossref] [PubMed]
- Zhang C, Liu X, Jin S, et al. Ferroptosis in cancer therapy: a novel approach to reversing drug resistance. Mol Cancer 2022;21:47. [Crossref] [PubMed]
- Wang H, Cheng Y, Mao C, et al. Emerging mechanisms and targeted therapy of ferroptosis in cancer. Mol Ther 2021;29:2185-208. [Crossref] [PubMed]
- Battaglia AM, Chirillo R, Aversa I, et al. Ferroptosis and Cancer: Mitochondria Meet the "Iron Maiden" Cell Death. Cells 2020;9:1505. [Crossref] [PubMed]
- Piska K, Gunia-Krzyżak A, Koczurkiewicz P, et al. Piperlongumine (piplartine) as a lead compound for anticancer agents - Synthesis and properties of analogues: A mini-review. Eur J Med Chem 2018;156:13-20. [Crossref] [PubMed]
- Avila-Carrasco L, Majano P, Sánchez-Toméro JA, et al. Natural Plants Compounds as Modulators of Epithelial-to-Mesenchymal Transition. Front Pharmacol 2019;10:715. [Crossref] [PubMed]
- Wang Z, Li Y, Wang D, et al. Ferroptosis molecular inducers: A future direction for malignant tumor chemotherapy. Biocell 2022;46:1599-611. [Crossref]
- Lv F, Deng M, Bai J, et al. Piperlongumine inhibits head and neck squamous cell carcinoma proliferation by docking to Akt. Phytother Res 2020;34:3345-58. [Crossref] [PubMed]
- Mukha A, Kahya U, Linge A, et al. GLS-driven glutamine catabolism contributes to prostate cancer radiosensitivity by regulating the redox state, stemness and ATG5-mediated autophagy. Theranostics 2021;11:7844-68. [Crossref] [PubMed]
- Shen YA, Chen CL, Huang YH, et al. Inhibition of glutaminolysis in combination with other therapies to improve cancer treatment. Curr Opin Chem Biol 2021;62:64-81. [Crossref] [PubMed]
- Yang WH, Qiu Y, Stamatatos O, et al. Enhancing the Efficacy of Glutamine Metabolism Inhibitors in Cancer Therapy. Trends Cancer 2021;7:790-804. [Crossref] [PubMed]
- Ishitsuka K, Hideshima T, Hamasaki M, et al. Honokiol overcomes conventional drug resistance in human multiple myeloma by induction of caspase-dependent and -independent apoptosis. Blood 2005;106:1794-800. [Crossref] [PubMed]
- Ye W, Huang Q, Tang T, et al. Synergistic effects of piperlongumine and gemcitabine against KRAS mutant lung cancer. Tumori 2021;107:119-24. [Crossref] [PubMed]
- Qian J, Xu Z, Zhu P, et al. A Derivative of Piperlongumine and Ligustrazine as a Potential Thioredoxin Reductase Inhibitor in Drug-Resistant Hepatocellular Carcinoma. J Nat Prod 2021;84:3161-8. [Crossref] [PubMed]
- Song Y, Yang H, Lin R, et al. The role of ferroptosis in digestive system cancer. Oncol Lett 2019;18:2159-64. [Crossref] [PubMed]
- Tang D, Chen X, Kang R, et al. Ferroptosis: molecular mechanisms and health implications. Cell Res 2021;31:107-25. [Crossref] [PubMed]
- Basit F, van Oppen LM, Schöckel L, et al. Mitochondrial complex I inhibition triggers a mitophagy-dependent ROS increase leading to necroptosis and ferroptosis in melanoma cells. Cell Death Dis 2017;8:e2716. [Crossref] [PubMed]
- Lei G, Mao C, Yan Y, et al. Ferroptosis, radiotherapy, and combination therapeutic strategies. Protein Cell 2021;12:836-57. [Crossref] [PubMed]
- Yamaguchi Y, Kasukabe T, Kumakura S. Piperlongumine rapidly induces the death of human pancreatic cancer cells mainly through the induction of ferroptosis. Int J Oncol 2018;52:1011-22. [Crossref] [PubMed]
- Zhang S, Hu R, Geng Y, et al. The Regulatory Effects and the Signaling Pathways of Natural Bioactive Compounds on Ferroptosis. Foods 2021;10:2952. [Crossref] [PubMed]
- Nie Q, Hu Y, Yu X, et al. Induction and application of ferroptosis in cancer therapy. Cancer Cell Int 2022;22:12. [Crossref] [PubMed]
- Ge C, Zhang S, Mu H, et al. Emerging Mechanisms and Disease Implications of Ferroptosis: Potential Applications of Natural Products. Front Cell Dev Biol 2022;9:774957. [Crossref] [PubMed]
- Wang X, Wang Y, Li Z, et al. Regulation of Ferroptosis Pathway by Ubiquitination. Front Cell Dev Biol 2021;9:699304. [Crossref] [PubMed]
- Gan B. Mitochondrial regulation of ferroptosis. J Cell Biol 2021;220:e202105043. [Crossref] [PubMed]
- Zhang L, Jia R, Li H, et al. Insight into the Double-Edged Role of Ferroptosis in Disease. Biomolecules 2021;11:1790. [Crossref] [PubMed]
- Skouta R, Dixon SJ, Wang J, et al. Ferrostatins inhibit oxidative lipid damage and cell death in diverse disease models. J Am Chem Soc 2014;136:4551-6. [Crossref] [PubMed]
- Lang L, Wang F, Ding Z, et al. Blockade of glutamine-dependent cell survival augments antitumor efficacy of CPI-613 in head and neck cancer. J Exp Clin Cancer Res 2021;40:393. [Crossref] [PubMed]
- Liu Y, Yang Y, Jiang L, et al. High Expression Levels of SLC38A1 Are Correlated with Poor Prognosis and Defective Immune Infiltration in Hepatocellular Carcinoma. J Oncol 2021;2021:5680968. [Crossref] [PubMed]
- Cao X, Li Y, Wang Y, et al. Curcumin suppresses tumorigenesis by ferroptosis in breast cancer. PLoS One 2022;17:e0261370. [Crossref] [PubMed]
- Kung FP, Lim YP, Chao WY, et al. Piperlongumine, a Potent Anticancer Phytotherapeutic, Induces Cell Cycle Arrest and Apoptosis In Vitro and In Vivo through the ROS/Akt Pathway in Human Thyroid Cancer Cells. Cancers (Basel) 2021;13:4266. [Crossref] [PubMed]