Down-regulation of CX43 expression by miR-1 inhibits the proliferation and invasion of glioma cells
Highlight box
Key findings
• Down-regulation of CX43 expression by miR-1 inhibited the infiltration and growth of glioma cells and further promoted the apoptosis of glioma cells by regulating CX43 expression.
What is known and what is new?
• Connexin 43 (CX43) makes glioblastoma resistant to temozolomide, the first-line chemotherapy drug.
• MicroRNA-1 may be a new marker of regulating CX43 expression.
What is the implication, and what should change now?
• Although the results of this study suggest that the CX43 gene plays an important role in the growth and development of malignant glioma cells, whether this gene can be an optimal target for malignant glioma gene therapy remains to be further investigated.
Introduction
Glioma accounts for approximately 46% of intracranial tumors, with an incidence rate of 3–10/100,000 (1-3). As glioma cells can proliferate infinitely and are extremely invasive, they exhibit characteristics including rapid and invasive growth as well as the ability to easily invade the surrounding normal brain tissue (4). Moreover, it is usually difficult to remove them completely, and the postoperative recurrence rate is high (5). The survival time of patients is short, the mortality rate is high, and the prognosis is poor. Glioma is the most common primary tumor of the central nervous system (CNS), accounting for about 40–60% (6-8). At present, the main treatment of glioma is tumor resection, followed by radiotherapy and chemotherapy. Owing to the heterogeneity of the mass, the unbounded combination of the mass and normal tissue, and the high invasiveness of glioma cells, the prognosis is poor, and postoperative recurrence is common (9). Therefore, there is an urgent need to explore new concepts and methods as well as novel molecular targets for the treatment of glioma. Many connexin (CX) molecules have been explored in the CNS: CX30, CX32, CX36, and CX43 in neurons. CX30, CX40, CX43 and CX45 in astrocytes; CX32, CX36 and CX43 in microglia; CX26, CX32, CX29, CX36 and CX47 in oligodendrocytes. Although the large family consists of connexins, CX43 is highly expressed in patients with glioblastoma tissue and seems to be a marker of glioma that distinguishes it from other types of brain cancer, such as oligodendrocyte. CX43 acts like a tumor suppressor, leading to dedifferentiation of cancer cells and inhibiting metastasis, thereby affecting tumor progression.
Gap junction (GJ) channels are formed by the pairwise docking of two adjacent cells through linkers (10). Under physiological conditions, the intercellular current, ion, and molecular weight are allowed to be less than 1.2 Ku molecules, with a diameter <10 nm, and for second messenger material to pass through (11). CX43 is the most abundant CX expressed in the CNS and is mainly distributed in astrocytes. A previous study has shown that CX43 is a tumor suppressor gene that can reverse the phenotypic transformation of cancer cells, such as breast cancer and neurotic glioma cells (12). Although the CX43 surface reach level change is not the initiating factor of glioma, the CX43 surface reach level is related to the pathological type and malignant degree of glioma.
CX43 is involved in tumor cell proliferation, migration, and adhesion, and has thus become a potentially important target for the treatment of glioma (13). Cytokines can maintain the rapid growth of tumor cells and promote their dissemination and metastasis so that tumor cells continue to proliferate, metastasize, and invade normal tissues. Due to gene mutations, most tumor cells overexpress epidermal growth factor (EGF), insulin-like growth factor (IGF), and platelet-derived growth factor (PDGF), as well as other growth factors and their receptors. Signaling of these factors is mainly through the mitogen-activated protein kinase (MAPK) signaling pathway, which is transmitted to the nucleus and induces a hyperproliferative response (14). MAPK is an important intracellular information transfer substance that is involved in the physiological and pathological processes of cell proliferation, differentiation, and apoptosis (15). Numerous studies have shown that MAPK can transmit membrane receptor stimulation signals to target sites in the nucleus and regulate cell gene transcription and protein synthesis (16-18). Furthermore, it also is the core loop segment of many extracellular pro-cell proliferation signal transduction pathways. The extracellular regulated protein kinases (ERK)1/2 pathway plays an important role in cell proliferation response caused by growth factor-related stimulation, while the p38MAPK pathway, which participates in the stress response, is mainly activated and regulated by damaging factors, such as ultraviolet light, hydrogen peroxide, and proinflammatory cytokines (16-18). Vascular endothelial cells can absorb miR-1 from glioma exosomes and enhance angiogenesis. On the contrary, overexpression of miR-1 in glioma cells can inhibit the proliferation and migration of human microvascular endothelial cells and the expression of vascular endothelial growth factor, a key factor of angiogenesis, in cell co-culture experiments. In conclusion, the regulation of miRNA on angiogenesis of glioma may indirectly affect the sensitivity of glioma to chemotherapy. Tumor-associated macrophages are another important cell component in glioma microenvironment.
Gap junction intercellular communication (GJIC) is closely related to tumors (19). The mutation, expression, or intracellular localization changes of CXs are found in most tumor cells, and the ability of GJIC is reduced or lost. As an important component of GJIC, CX43 regulates the function of GJIC to a large extent. Some researchers have pointed out that higher malignant degrees of brain glioma are indicative of weaker GJIC function and reduced CX43 expression. Transfection of CX43 into human glioma cells, U251 and T98G, significantly inhibits cell proliferation, suggesting that CX43 may be a tumor suppressor gene (20). CX43 is a promising therapeutic target for glioma, and therefore, investigating the regulation of CX43 is critical. The present study aimed to determine whether microRNA (miR)-1 could regulate CX43 in glioma cells and affect its invasive growth. We present the following article in accordance with the MDAR reporting checklist (available at https://tcr.amegroups.com/article/view/10.21037/tcr-22-2318/rc).
Methods
Source of glioma cell lines
Glioma cell lines was purchased from company (Procell Life Science&Technology Co., Ltd., Wuhan, China).
Stable cell lines were screened
Cells were seeded into six-well plates at 5×107 cells·L−1 and cultured for 24 h for transfection. Solution A was prepared as follows: 240 µL serum free culture base + 10 µL Lipofectamine 2000 in a total volume of 250 µL, which was incubated for 5 min at room temperature. As for Solution B, 220 µL serum-free medium + 30 µL plasmid (130 mg·L−1) was added to each well (total volume =250 µL). Solutions A and B were thoroughly mixed and incubated at chamber temperature for 20 min. The cells in the six-well plate were washed twice with phosphate-buffered saline (PBS) and 2 mL serum-free medium was added. Next, 500 µL of solution A/B mixture was added, and the culture plate was shaken and mixed. After 6 h, the volume fraction of replacement was 0. Dulbecco’s Modified Eagle Medium (DMEM) medium with 1 fetal bovine serum (FBS) was cultured for 48 h, and then DMEM medium containing G418 (800 mg·L−1) was replaced for screening. Fresh medium containing G418 was used every 3 days to replace the medium containing a large number of dead cells. When the resistant cells became overgrown, the cells were subcultured and frozen for preservation.
Cell culture
U251 cells were cultured in DMEM medium containing 10% FBS in incubators containing 5% CO2 at 37 °C in saturated humidity. Cell planking and transfection was performed as follows: 293T cells in the logarithmic growth phase and in a good growth state were digested with 0.25% trypsin. After calculation by cell counting, they were seeded into 24-well plates at a density of 6×104 cells/well and spread for 18–24 h (try not to exceed 24 hours, otherwise transfection efficiency will be affected). Cell length to 70–90% transfection (cell density that is too thin or too dense will affect the transfection efficiency).
Real-time quantitative polymerase chain reaction (RT-qPCR) experiment
We extracted total RNA using TRIzol (Invitrogen) or a miRNeasy Kit (QIAGEN, Cat, 217004). For mRNA detection, cDNA was obtained using a reverse transcription system kit (Promega) with oligo-DT primers. Quantitative reverse transcription polymerase chain reaction (qRT-PCR) assays were performed in a one-step + system (Applied Biosystems, America) using SYBRGreenPCRMas (Applied Biosystems) with kit according to the standard protocols, and glyceraldehyde-3-phosphate dehydrogenase (GAPDH) was selected as an endogenous control. A miRNA assay was designed with miR-specific stem-loop primers for reverse transcription, and U6RNA was used as an endogenous miRNA control. The primer design is shown in Table 1.
Table 1
Primers | Primer sequences (5'→3') | Source of primers |
---|---|---|
hsa CX43-103-F | AGGAGTTCAATCACTTGGCG | Synthetic by manual design |
hsa CX43-103-R | ACCTTGTCAAGGAGTTTGCC | Synthetic by manual design |
GAPDH-127F(H) | CCAGGTGGTCTCCTCTGA | Synthetic by manual design |
GAPDH-127R(H) | GCTGTAGCCAAATCGTTGT | Synthetic by manual design |
CX, connexin; GAPDH, glyceraldehyde-3-phosphate dehydrogenase.
Westernblot (WB) experimental analysis
WB experimental analysis was performed according to the standard protocols. Briefly, total cell lysates were prepared in sodium dodecyl sulfate buffer. We loaded equal amounts of protein onto sodium dodecyl sulfate-polyacrylamide gel electrophoresis for curing, and then transferred the protein to a polyvinylidene fluoride membrane. After dilution, the following primary antibody ratio was added: anti-MMP2 (Abcam, America), anti-MMP3 (Abcam, 1:2,000), anti-MMP2 (Abcam, 1:2,000), anti-gum, (Santa Cruz Biotechnology, 1:500), anti-collagen II (well, America, 1:1,000), anti-collagen I (Millip, America,1:1,000), and anti-GAPDH (Abcam, America, 1:5,000).
Dual luciferase gene testing
The dual-luciferase gene was used to detect whether the miR-1 gene was the direct target gene of CX43. Forty-eight hours after transfection, the cells were harvested and lysed. Luciferase activity was measured using the luciferase detection Kit (K801-200, USA) and the Glomax20/20 Luminescence Kit (Madison, USA). In addition, each experiment was repeated three times. The relative light unit (RLU) was measured after gun beating or mixed in other appropriate ways, and the expression of one gene in the U251 cell samples was further quantitatively detected by qPCR assay.
Cell transfection assay
The cells were isolated with 0.25% trypsin, subcultured at a ratio of 1:2 or 1:3, cultured in DMEM supplemented with 10% FBS, and incubated in a 5% CO2 incubator at 14,837 °C. The cells were treated with 0.25% trypsin for 30 seconds every 2 days. After removing the supernatant, we suspended the cells in 1 mL of fresh complete medium and packed them in two sterile culture flyers at a ratio of 1:2 to 1:5 and supplemented the medium to 4 mL before the cells were cultured in a 5% CO2 incubator at 37 °C. When the cells reached the logarithmic growth stage, they were assigned to the simulated normal cells, CX43-WT miR-NC, miR-1-3p, CX43-Mut miR-NC, and miR-1-3p groups. The cells were seeded in six-well plates 24 h before transfection. When cells reached 50% confluence, we transfected them using Liposome 2000 (Carlsbad, USA). The medium was changed after 6 hours of incubation, and the cells were collected after 48 hours of incubation to facilitate further experiments.
Statistical analysis
All experiments in this study were repeated at least three times, and experimental data were expressed as mean ± standard deviation (SD). We used the Student’s t-test to assess continuous data between the two groups and the Kruskal-Wallis test was adopted for multiple group comparisons. In addition, statistical analyses were performed using GraphPad Prism 8 and R software (Version 3.6.1, America). P<0.05 was considered to indicate a statistically significant difference.
Results
Analysis of the growth and proliferation cycle of glioma cells
In this study, flow cytometry showed that the miR-1-3p mimics model of U251 cells had a certain inhibitory effect on cell proliferation, while the miR-1-3p inhibitor model of U251 cells exhibited a certain proliferation-promoting effect (Figure 1).
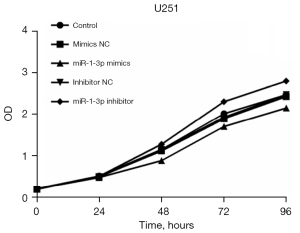
Analysis of brain glioma cell apoptosis
The experimental results showed that compared with the mimics normal control (NC) group, the apoptosis rate of the miR-1-3p mimics group was decreased (Table 2), while that of the miR-1-3p inhibitor group was increased compared with the inhibitor NC group (Figures 2 and 3).
Table 2
Group | Control | mimics NC | miR-1-3p mimics | Inhibitor NC | miR-1-3p inhibitor |
---|---|---|---|---|---|
Cell apoptosis (%) | 5.24 | 11.44 | 5.53 | 4.56 | 8.81 |
mimics NC, mimics normal control; miR, microRNA.
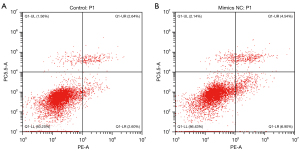
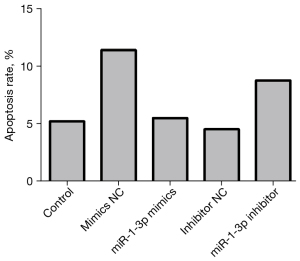
Transwell assay was used to detect the invasiveness of cell lines
Transfection efficiency was usually measured by flow cytometry at 48 h after transfection. Through fluorescence (FL), 1 green-fluorescent protein (GFP) (pTTo/GFPq, NRC, BRI; 5% of the total plasmid DNA) was applied to detect the transfected cells. Dead cells were stained with 2 µg/mL propidium iodide. The miR-1-3p mimics model of U251 cells exerted an inhibitory effect on the invasion ability of cells (Table 3), whereas the miR-1-3p inhibitor model of U251 cells exhibited an invasion-promoting effect (Figures 4 and 5).
Table 3
Group | Control | mimics NC | miR-1-3p mimics | Inhibitor NC | miR-1-3p inhibitor |
---|---|---|---|---|---|
Invasiveness of cell lines (mean ± standard deviation) | 535.667±31.50 | 529.667±25.81 | 444.333±18.15 | 513.333±17.04 | 638.667±9.71 |
mimics NC, mimics normal control; miR, microRNA.

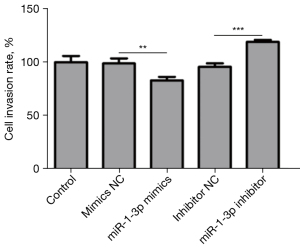
Analysis of the dual-luciferase test results
The dual-luciferase assay was used to detect the regulatory relationship between gene elements related to plasmid vectors. The results showed that the luciferase activity of miR-1-3p decreased significantly compared with miR-NC in the CX43-WT group. However, in the CX43-mut group, the luciferase activity of miR-1-3p did not change compared with miR-NC. Thus, it was concluded that miR-1-3p has a targeted relationship with the CX43 gene (Figure 6).
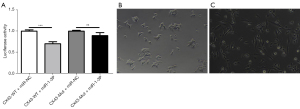
qRT-PCR and western blotting were applied to detect the expression of miR-1 and CX43
Our results showed that the expression of miR-1-3P-mimics in the CX43 genome was decreased compared with that in the mimics-NC control group. Also, compared with the inhibitor-NC control group, the expression of the miR-1-3P-inhibitor group was up-regulated (Figure 7).

Analysis of the cell histochemical experimental results
Immunohistomorphological observation is the basis of pathological diagnosis. Common immunohistochemical markers include GFAP, Olig2, EMA, EGFRvIII, KI-67, ATRX, TP53, RELA, and BRAFV600E. The most recent version of the CNS tumor classification has changed the traditional morphological classification method of glioma and proposed a comprehensive classification of glioma based on its histopathological and molecular characteristics. The key genetic materials related to the development and progression of glioma were included in this classification, including IDH1/2 mutation, chromosome 1P/19q co-deletion, and histone H3K27M mutation (Figure 8).
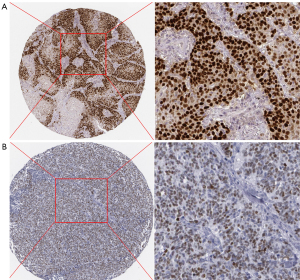
Discussion
Glioma is a common malignant intracranial tumor with a high incidence. It is difficult to achieve complete surgical resection of glioma, and its degree of malignancy is increasing (21). CX43 (a tumor suppressor gene that inhibits tumor cell growth) and PCNA (an indicator of tumor cell proliferation) have been recognized as important by many scholars in this field (22). Numerous tumors exhibit decreased or absent CX43 gene expression and are associated with disrupted GJ communication. Also, up-regulated expression of this gene can inhibit the growth of tumor cells (23). Proliferating cell nuclear antigen (PCNA) is a nuclear protein that is essential for DNA synthesis in eukaryotic cells. It is mainly expressed in proliferative cells and has obvious periodicity. The periodicity is consistent with DNA synthesis, and its expression increased with the degree of tumor malignancy. Therefore, PCNA can be used as an index to explore cell proliferation status (24). Studies have been conducted on the expression of the CX43 gene in glioma both at home and abroad; however, the relationship between CX43 gene expression and cell proliferation in human glioma has not yet been reported. We applied immunohistochemistry to detect the CX43 protein and in situ molecular hybridization to detect the mRNA expression of CX43 in glioma. At the same time, PCNA was used as an indicator of cell proliferation to analyze the relationship between CX43 gene expression and glioma proliferation and to explore the mechanism of CX43 gene expression and glioma (25-27).
The CX gene family encodes proteins that constitute the basic structural and functional units of GJs, which mediate intercellular communication (28). In many tumors, CX gene expression is decreased or absent and gap junction communication (GJ1C) is disrupted. As early as 1966, Loewenstein and Kanno discovered a lack of intercellular electrical coupling in tumor cells; that is, a breakdown of intercellular communication at GJs. Later studies confirmed that GJ communication defects were prevalent in tumor and transformed cells, and GJIC recovery was associated with the control of tumor growth and the inhibition of phenotypic transformation. Since then, the role of the CX gene and GJIC in the process of tumor cell degeneration has been directly confirmed by the isolation of CX and the cloning of its gene (29). Some members of the CX gene family have the ability to inhibit cell growth and coordinate the growth of a single cell with adjacent cells, exhibiting the characteristics of tumor suppressor genes. Thus, this is considered to be a family of tumor suppressor genes (30).
CX43 is encoded by a 2,768 bp span of DNA. The cDNA contains a 1,146 bp open reading frame, which is named accordingly because it encodes a single peptide with a molecular weight of 43 kD and contains 378 amino acids (31). A recent study has found that the decrease or loss of CX43 expression is closely related to the occurrence and development of glioma, lung cancer, breast cancer, cervical cancer, and other tumors (32). Domestic literature has reported that CX43 is highly expressed in the epithelial cell membranes of normal gastric and laryngeal mucosa, while its expression is reduced or absent in gastric and laryngeal cancer cells, and it is mostly located in the cytoplasm. Moreover, a relevant study has detected the expression of CX43 in breast cancer specimens and found that CX43 is the main GJ protein in normal breast tissues but not in carcinoma in situ or invasive carcinoma. CX43 deficiency is also a common feature of human breast cancer tissues (33).
The expression of the CX gene and its encoded protein in human glioma can be decreased or deleted, as is the case in several other malignant tumors. CX43 change is not the initiating factor of glioma (34). Therefore, it is believed that decreased CX43 expression may cause glioma to progress from a low to a high malignant degree or increase tumor aggressiveness. Recently, immunohistochemistry and western blotting were used to report the expressions of CX43 and CX26 in various tumors (35). CX43 was found to be expressed not only in astrocytoma but also in oligodendroglioma, ganglioglioma, meningioma, and medulloblastoma, indicating that the cells were dedifferentiated during tumor development.
In recent years, scholars have devoted themselves to studying the relevant factors of the occurrence and development of various malignant tumors (36-40). This can provide a theoretical basis for the prevention and treatment of malignant tumors. The CX gene and its encoded protein are widely expressed in nervous system tumors, especially in gliomas of all levels. However, the expression level require further study. Although the results of this study suggest that the CX43 gene plays an important role in the growth and development of malignant glioma cells, whether this gene can be an optimal target for malignant glioma gene therapy remains to be further investigated.
Conclusions
The down-regulation of CX43 expression provides a new target for us to treat glioma, but it still needs further clinical research to confirm.
Acknowledgments
Funding: This study was supported by
Footnote
Reporting Checklist: The authors have completed the MDAR reporting checklist. Available at https://tcr.amegroups.com/article/view/10.21037/tcr-22-2318/rc
Data Sharing Statement: Available at https://tcr.amegroups.com/article/view/10.21037/tcr-22-2318/dss
Conflicts of Interest: All authors have completed the ICMJE uniform disclosure form (available at https://tcr.amegroups.com/article/view/10.21037/tcr-22-2318/coif). The authors have no conflicts of interest to declare.
Ethical Statement: The authors are accountable for all aspects of the work in ensuring that questions related to the accuracy or integrity of any part of the work are appropriately investigated and resolved.
Open Access Statement: This is an Open Access article distributed in accordance with the Creative Commons Attribution-NonCommercial-NoDerivs 4.0 International License (CC BY-NC-ND 4.0), which permits the non-commercial replication and distribution of the article with the strict proviso that no changes or edits are made and the original work is properly cited (including links to both the formal publication through the relevant DOI and the license). See: https://creativecommons.org/licenses/by-nc-nd/4.0/.
References
- Gusyatiner O, Hegi ME. Glioma epigenetics: From subclassification to novel treatment options. Semin Cancer Biol 2018;51:50-8. [Crossref] [PubMed]
- Nicholson JG, Fine HA. Diffuse Glioma Heterogeneity and Its Therapeutic Implications. Cancer Discov 2021;11:575-90. [Crossref] [PubMed]
- Peng Z, Liu C, Wu M. New insights into long noncoding RNAs and their roles in glioma. Mol Cancer 2018;17:61. [Crossref] [PubMed]
- Malta TM, de Souza CF, Sabedot TS, et al. Glioma CpG island methylator phenotype (G-CIMP): biological and clinical implications. Neuro Oncol 2018;20:608-20. [Crossref] [PubMed]
- Miller AM, Shah RH, Pentsova EI, et al. Tracking tumour evolution in glioma through liquid biopsies of cerebrospinal fluid. Nature 2019;565:654-8. [Crossref] [PubMed]
- de Blank P, Bandopadhayay P, Haas-Kogan D, et al. Management of pediatric low-grade glioma. Curr Opin Pediatr 2019;31:21-7. [Crossref] [PubMed]
- Hervey-Jumper SL, Berger MS. Insular glioma surgery: an evolution of thought and practice. J Neurosurg 2019;130:9-16. [Crossref] [PubMed]
- Bai J, Varghese J, Jain R. Adult Glioma WHO Classification Update, Genomics, and Imaging: What the Radiologists Need to Know. Top Magn Reson Imaging 2020;29:71-82. [Crossref] [PubMed]
- Poff A, Koutnik AP, Egan KM, et al. Targeting the Warburg effect for cancer treatment: Ketogenic diets for management of glioma. Semin Cancer Biol 2019;56:135-48. [Crossref] [PubMed]
- Hao Z, Hu S, Liu Z, et al. Circular RNAs: Functions and Prospects in Glioma. J Mol Neurosci 2019;67:72-81. [Crossref] [PubMed]
- Norouzi M. Gold Nanoparticles in Glioma Theranostics. Pharmacol Res 2020;156:104753. [Crossref] [PubMed]
- Chatwin HV, Cruz Cruz J, Green AL. Pediatric high-grade glioma: moving toward subtype-specific multimodal therapy. FEBS J 2021;288:6127-41. [Crossref] [PubMed]
- Ghantasala S, Gollapalli K, Epari S, et al. Glioma tumor proteomics: clinically useful protein biomarkers and future perspectives. Expert Rev Proteomics 2020;17:221-32. [Crossref] [PubMed]
- Ruff M, Kizilbash S, Buckner J. Further understanding of glioma mechanisms of pathogenesis: implications for therapeutic development. Expert Rev Anticancer Ther 2020;20:355-63. [Crossref] [PubMed]
- Jin W, Fatehi M, Abhishek K, et al. Artificial intelligence in glioma imaging: challenges and advances. J Neural Eng 2020;17:021002. [Crossref] [PubMed]
- Wei X, Xiao B, Wang L, et al. Potential new targets and drugs related to histone modifications in glioma treatment. Bioorg Chem 2021;112:104942. [Crossref] [PubMed]
- Bao Z, Wang Y, Wang Q, et al. Intratumor heterogeneity, microenvironment, and mechanisms of drug resistance in glioma recurrence and evolution. Front Med 2021;15:551-61. [Crossref] [PubMed]
- Zhao N, Zhang J, Zhao Q, et al. Mechanisms of Long Non-Coding RNAs in Biological Characteristics and Aerobic Glycolysis of Glioma. Int J Mol Sci 2021;22:11197. [Crossref] [PubMed]
- Epifantseva I, Shaw RM. Intracellular trafficking pathways of CX43 gap junction channels. Biochim Biophys Acta Biomembr 2018;1860:40-7. [Crossref] [PubMed]
- Wu L, Zheng Y, Liu J, et al. Comprehensive evaluation of the efficacy and safety of LPV/r drugs in the treatment of SARS and MERS to provide potential treatment options for COVID-19. Aging (Albany NY) 2021;13:10833-52. [Crossref] [PubMed]
- Ai X, Yan J, Pogwizd SM. Serine-threonine protein phosphatase regulation of CX43 dephosphorylation in arrhythmogenic disorders. Cell Signal 2021;86:110070. [Crossref] [PubMed]
- Barnett SD, Asif H, Anderson M, et al. Novel Tocolytic Strategy: Modulating CX43 Activity by S-Nitrosation. J Pharmacol Exp Ther 2021;376:444-53. [Crossref] [PubMed]
- Mannell H, Kameritsch P, Beck H, et al. CX43 Promotes Endothelial Cell Migration and Angiogenesis via the Tyrosine Phosphatase SHP-2. Int J Mol Sci 2021;23:294. [Crossref] [PubMed]
- Golan K, Singh AK, Kollet O, et al. Bone marrow regeneration requires mitochondrial transfer from donor CX43-expressing hematopoietic progenitors to stroma. Blood 2020;136:2607-19. [Crossref] [PubMed]
- De Smet MA, Lissoni A, Nezlobinsky T, et al. CX43 hemichannel microdomain signaling at the intercalated disc enhances cardiac excitability. J Clin Invest 2021;131:137752. [Crossref] [PubMed]
- Wu DP, Zhou Y, Hou LX, et al. CX43 deficiency confers EMT-mediated tamoxifen resistance to breast cancer via c-Src/PI3K/Akt pathway. Int J Biol Sci 2021;17:2380-98. [Crossref] [PubMed]
- Yu M, Lin Z, Tian X, et al. Downregulation of CX43 reduces cisplatin-induced acute renal injury by inhibiting ferroptosis. Food Chem Toxicol 2021;158:112672. [Crossref] [PubMed]
- Lissoni A, Hulpiau P, Martins-Marques T, et al. RyR2 regulates CX43 hemichannel intracellular Ca2+-dependent activation in cardiomyocytes. Cardiovasc Res 2021;117:123-36. [Crossref] [PubMed]
- Martins-Marques T, Catarino S, Gonçalves A, et al. EHD1 Modulates CX43 Gap Junction Remodeling Associated With Cardiac Diseases. Circ Res 2020;126:e97-e113. [Crossref] [PubMed]
- Zhang B, Liu C, Bao L, et al. CX43 silencing inhibits mechanical stress-induced apoptosis in mouse articular chondrocyte. Zhong Nan Da Xue Xue Bao Yi Xue Ban 2019;44:28-34. [PubMed]
- Wu L, Zheng Y, Ruan X, et al. Long-chain noncoding ribonucleic acids affect the survival and prognosis of patients with esophageal adenocarcinoma through the autophagy pathway: construction of a prognostic model. Anticancer Drugs 2022;33:e590-603. [Crossref] [PubMed]
- Strauss RE, Mezache L, Veeraraghavan R, et al. The CX43 Carboxyl-Terminal Mimetic Peptide αCT1 Protects Endothelial Barrier Function in a ZO1 Binding-Competent Manner. Biomolecules 2021;11:1192. [Crossref] [PubMed]
- Costa E, Okesola BO, Thrasivoulou C, et al. CX43 mediates changes in myofibroblast contraction and collagen release in human amniotic membrane defects after trauma. Sci Rep 2021;11:16975. [Crossref] [PubMed]
- Chen R, Chen Y, Yuan Y, et al. CX43 and AKAP95 regulate G1/S conversion by competitively binding to cyclin E1/E2 in lung cancer cells. Thorac Cancer 2020;11:1594-602. [Crossref] [PubMed]
- Gao RJ, Zhang AM, Jia QH, et al. The promoting role of CX43 on the proliferation and migration of arterial smooth muscle cells for angiotensin II-dependent hypertension. Pulm Pharmacol Ther 2021;70:102072. [Crossref] [PubMed]
- Teng D, Xia S, Hu S, et al. miR-887-3p Inhibits the Progression of Colorectal Cancer via Downregulating DNMT1 Expression and Regulating P53 Expression. Comput Intell Neurosci 2022;2022:7179733. [Crossref] [PubMed]
- Chen Y, Wang J, Zhang X, et al. Correlation between apparent diffusion coefficient and pathological characteristics of patients with invasive breast cancer. Ann Transl Med 2021;9:143. [Crossref] [PubMed]
- Qi A, Li Y, Yan S, et al. Effect of postoperative chemotherapy on blood glucose and lipid metabolism in patients with invasive breast cancer. Gland Surg 2021;10:1470-7. [Crossref] [PubMed]
- He XC, Chen HY, Qiu Y, et al. Associations of iron status with breast cancer risk factors in adult women: Findings from National Health and Nutrition Examination Survey 2017-2018. J Trace Elem Med Biol 2021;68:126867. [Crossref] [PubMed]
- Qi A, Li Y, Sun H, et al. Incidence and risk factors of sexual dysfunction in young breast cancer survivors. Ann Palliat Med 2021;10:4428-34. [Crossref] [PubMed]
(English Language Editor: A. Kassem)