This article has an erratum available at: http://dx.doi.org/10.21037/tcr-2023-02 the article has been update on 2023-09-18 at here.
PAK4-relevant proliferation reduced by cell autophagy via p53/mTOR/p-AKT signaling
Highlight box
Key findings
• PAK4 downregulation is association with autophagy in HepG2 cells.
What is known and what is new?
• The proliferation is reduced by cell cycle arrest in PAK4-knockdown HepG2 cells.
• The proliferation is reduced by cell autophagy in PAK4-knockdown HepG2 cells.
What is the implication, and what should change now?
• PAK4 involves in cell autophagy besides of cell cycle. Cell autophagy and relative signaling molecules could be considered as biomarkers for PAK4 block in cancer therapy.
Introduction
The p21-activated kinases (PAKs) are serine/threonine kinases and usually classified into Group I (PAK1–3) and II (PAK4–6). PAK4 involves in a series of malignant phenotypes such as abnormal growth, cancer stem cells, epithelial-mesenchymal transition, and drug-resistance (1-4). Its inhibitors are shown in recent research to interfere with the progression of carcinoma cell cycle. The inhibitor KY04031 induces cell cycle arrest in A549 at G1/S phase (5). KPT-9274, a dual PAK4/nicotinamide phosphoribosyl transferase (NAMPT) inhibitor, fosters reduction of G2/M transition and induction of apoptosis in renal cancer cells (6). An overexpressed orphan gene INKA1, interfering with nuclear localization of PAK4, increases the proportion of leukemia stem cells in G0 by in acute myeloid leukemia (7). Silencing PAK4 by small interfering RNA (siRNA) induces a G2/M block in cancer cells because of the dysfunction of Ran GTPase and polo-like kinase 1 as its substrates (8,9). These observations imply that PAK4 modulates cell cycle in multiple phases for cancer cell growth.
p53, a molecule upstream to PAK4 by interacting with p21 (10), protects cells via cell-cycle-surveillance on G1/S phase (11). Therefore, p53-mediated G1/S arrest is speculated to impair proliferation in PAK4-blockdown cancer cells. However, like PAK4, p53-p21 pathway controls all checkpoints from DNA synthesis to cytokinesis including G1/S, G2/M, and spindle assembly during the cell cycle (11-13). Further, there is a mutual regulation between p53 and PAK4 because PAK4 is detected to phosphorylate directly p53, which not only attenuates p53 in transcriptional transactivation activity but also inhibits p53-mediated suppression of hepatocellular carcinoma (HCC) cell invasion (14). Therefore, these pieces of evidence make it easy to understand there remains mechanism underlying PAK4-relevant cell proliferation modulated by p53 besides the process of cell cycle.
Indeed, PAK-relative factors induced cancer cell cycle arrest but also the autophagy following being coupled to acetyltransferases (15). PAK inhibitors in meningioma cells induce cell death with increased autophagy-related protein levels and chromosomes-markers for mitotic catastrophe (16). The inhibition of potential PAK1 interactors revealed the importance of genes related to the autophagy in PAK1-mediated glioma cell proliferation (17). The autophagy is induced in neuroblastoma by targeting circRNA-195 to downregulate PAK2 expression (18). PAK1-knockout fuels autonomous autophagy under nutrient-limiting conditions in prostate cancer cells (19). It implies that a few PAK family members involve in cell autophagy besides of cell cycle although there is no evidence to connect PAK4 with autophagy.
Furthermore, the autophagy is performed by mammalian target of rapamycin (mTOR)/protein kinase B (AKT) signaling in a few studies. The activator of mTOR increases the expression of PAK1, p-PAK1 and decreases the ratio of LC3B2/LC3B1 in prostate cancer cells (20). Ivermectin-induced autophagy is accompanied with decreased PAK1 expression in breast cancer via the ubiquitination-mediated degradation pathway, which decreases the phosphorylation level of AKT, resulting in the blockade of the mTOR/AKT signaling pathway (21). The ubiquitination-mediated degradation of PAK1 blocks mTOR/AKT1 signaling pathway, leading to increased autophagic flux to treat lung cancer (22). In particular, Atg7 (autophagy gene)-deficient hepatocytes fail to repopulate the liver accompanied with downregulated PAK4 protein in a hepatic injury model, which is overcome by pharmacological mTOR inhibition (23).
Also, p53 is suggested to control cell autophagy via mTOR/AKT signaling. The p53-inhibited mTOR activates autophagy in cultured cells under stress (24) similar as rapamycin-suppressed mTOR (25), while the deficiency of p53 prevents fibroblast autophagy and noticeably increases the activity of mTOR in tumors (25,26). The suppressed autophagy with low expression of autophagic markers presents a decrease in mTOR’s kinase activity, i.e., insufficient phosphorylation for AKT in fibroblasts (25). Analogously, the impaired mTOR/AKT signaling induces autophagy in HeLa cells (27). The mTOR/AKT signaling similarly performs to autophagic flux in maintenance of stemness and differentiation potential of P19 embryonic carcinoma cells (28).
According to these observations, a simultaneous modulation is inferred for PAK4-relevant proliferation by autophagy and cell cycle, and mechanistically, p53, mTOR and AKT may involve in the modulation. We present the following article in accordance with the MDAR reporting checklist (available at https://tcr.amegroups.com/article/view/10.21037/tcr-22-2272/rc).
Methods
Cell culture and reagents
HepG2 (human hepatocarcinoma cell line) cells were obtained from American Type Culture Collection (ATCC). The cells were maintained in Dulbecco’s Modified Eagle’s Medium (DMEM) medium, which is supplemented with 10% fetal calf serum (FCS; Invitrogen, CA, USA), penicillin G (100 U/mL), and streptomycin (100 mg/mL). The cells of HepG2 were cultured in a 37 ℃ humidified incubator with 5% CO2 and periodically cultured with 0.25% weight/volume (w/v) trypsin solution.
Transient and stable PAK4-knockdown HepG2 cell lines
PAK4-RNAi were cloned into hU6-MCS-CMV-GFP-SV40-Neomycin vector and sequenced by Genechem Co.,Ltd (Shanghai, China). The shRNA-PAK4 sequence was 5’-GGATGAACGAGGAGCAGAT-3’ and 5’-CTTCATCAAGATTGGCGAG-3’, and shRNA-Control sequence was 5’-TTCTCCGAACGTGTCACGT-3’. The lentivirus containing PAK4-RNAi plasmid was acquired from Genechem Co.,Ltd. The sequences were same as above.
We transfected commercial PAK4-RNAi plasmid and lentivirus into HepG2 cells in a 24-well plate respectively, and selected steadily infected monoclonal cells with puromycin (2 µg/mL). Lentivirus infection ratio was continuously monitored through immunofluorescence (lentivirus labelled with green fluorescent protein; GFP) and confirmed by western blot.
Cell Counting Kit 8 (CCK-8) assay
As cell proliferation assay, 2×104 cells of 100 µL were seeded into each well in 96-well plates to culture for 24 h at 37 ℃. And the plates were incubated for 10 min at 37 ℃ after the 10 µL solution was added to each well. The optical density (OD) of each well was detected at 450 nm (Dojindo, Rockville, MD, USA).
Methyl thiazolyl tetrazolium (MTT) assay
The cell viability of HepG2 was evaluated with MTT assay. Briefly, HepG2 cells were digested and plated onto 96-well plates with 85% confluence. After 8 hours, we added 0.01 mL MTT of 5 mg/mL in PBS (phosphate buffered saline) solution, placed the plates in a brood chamber at 37 ℃ for 4 h, and replaced medium with 0.1 mL DMSO (dimethylsulfoxide). After incubation at 37 ℃ for 15 min, the OD of each group was measured at 570 nm using spectrophotometer (Bio-Rad, Hercules, CA, USA).
Cell cycle analysis
The trypsinized cells in suspension (2×106/mL) was fixed with 70% ice-cold ethanol for 12 h at 4 ℃. We incubated HepG2 cells in cold PBS containing RNase A (Takara, Japan) for 1 h at 37 ℃. Cells were treated with propidium iodide (PI) at a final concentration of 5 µg/mL (Beyotime Biotechnology, Shanghai, China), and incubated at room temperature for 25 min. Finally, cells were subjected to flow cytometry (FCM) and analyzed with Mod Fit LT (Verity Software House, ME, USA).
Immunofluorescence
Before cell seeding, a small 8 mm × 8 mm slide was placed in every well in a 24-well plate. When HepG2 cells approach approximately 60% confluence, cell slides were washed in PBS and fixed in 95% ethylalcohol for 30 min at room temperature. The cell slides were then incubated with primary antibody for 12 h at 4 ℃, followed by a brief wash thrice with PBS for 5 min and 1 h incubation at 37 ℃ with secondary fluorescent antibodies, IgG/Cy3 (bs-0295G-Cy3, Bioss, Beijing) and IgM/RBITC (bs-0368R-RBITC, Bioss, Beijing). Slides were rinsed in PBS for 5 min three times, and nucleus was stained with 1 mg/mL DAPI of 200 µL (Beyotime Biotechnology) for 15 min. After drying, the mounted slides were pictured with a fluorescence microscope. The mean immunofluorescent intensity was calculated for target proteins using the software ImageJ.
Western blot
The cell lysate for immunoblotting was extracted using radio-immuno precipitation assay (RIPA) lysis buffer (Cwbio, Beijing, China) with protease inhibitors (Roche, Germany) added. BCA Assay Kit (Cwbio) was used to quantify protein concentration. Protein sample mixed with a loading buffer (40 µg) was separated on sodium dodecyl sulfate-polyacrylamide gel electrophoresis (SDS-PAGE) for electrophoresis and transferred in a Tris-Glycine buffer to polyvinylidene fluoride (PVDF) membranes (Millipore, Danvers, USA). The PVDF membranes were blocked by 5% of defatted milk. The membranes were blocked and then incubated with specific primary antibodies for 12 h at 4 ℃. After washed thrice with Tris-buffered saline with Tween-20 (TBST), membranes were incubated with secondary antibody for 1 h at 37 ℃. Finally, immunoreaction signal was detected with enhanced chemiluminescence (ECL) solution (WBLUC0100, Millipore). Signal intensity of membranes was quantified using ImageJ software (Bio-Rad).
The antibody of anti-human PAK4 was purchased from Santa Cruz, California, USA, anti-Akt, anti-p-Akt, anti-mTOR, anti-LC3, anti-Tubulin, anti-glyceraldehyde-3-phosphate dehydrogenase (GAPDH), anti-p53 from Immuno Way, Texas, USA, and horseradish peroxidase-conjugated secondary antibodies from Gene Tex, Texas, USA.
Monodansylcadaverine (MDC) staining
HepG2 cells were cultured on coverslips in 24-well plates for 48 h as shControl, shPAK4, and positive control were administered by rapamycin groups. After the medium was discarded and cells rinsed with 1× wash buffer, 100 µL MDC (50 µmol/L, Sigma-Aldrich) was added into wells and protected from light for 45 mins. Cells were fixed with 4% paraformaldehyde for 15 min after being washed twice. The images were obtained by fluorescence photometry. Based on the mean density of fluorescence, the statistical analysis was performed among groups.
Statistical analysis
The data were normatively expressed as “mean ± SD” from 3 separate experiments at least. One-way analysis of variance (ANOVA) was used to compare differences among multiple experiment groups, and t-test for pairs. Calculations were performed using SPSS 22.0 statistical software. Statistical significance was determined by P value less than 0.05.
Ethical statement
The study was conducted in accordance with the Declaration of Helsinki (as revised in 2013). Institutional ethical approval and informed consent were waived.
Results
Transient and stable knockdown of PAK4 inhibit proliferation in HepG2 cells
Successful construction of transient PAK4-knockdown HepG2 was verified by western blot (P<0.05; Figure 1A). To investigate whether PAK4 was associated with cell proliferation in HepG2 cells, we transfected cells with PAK4-RNAi plasmid and performed MTT assay by OD value detection on day 1, 2, 3, 4 and 5. We found that there were no statistical differences among group shPAK4, shControl, and Control on day 1 and 2. Meanwhile, the proliferation of cells in group shPAK4 exhibited an obvious reduction compared with group shControl and Control on day 3, 4 and 5 (P<0.05; Figure 1A).
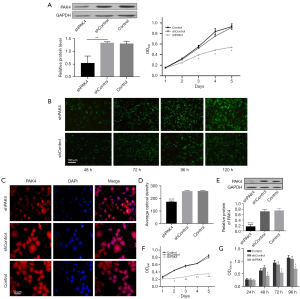
Further, we established stable shPAK4 and shControl cell lines using Lentiviral Expression System. HepG2 cells were briefly infected with lentiviral particles containing shPAK4 or scramble sequences. Lentivirus infection ratio was continuously monitored by immunofluorescence (lentivirus labelled with GFP) at hour 48, 72, 96 and 120. Only at hour 96 and 120, the cells showed maximum GFP fluorescence intensity, demonstrating that lentiviral particles were fully integrated into chromosomes of HepG2 (Figure 1B).
PAK4 was identified for its expression and subcellular localization by immunofluorescence with anti-PAK4 primary antibody and fluorescent-dye conjugated secondary antibody (red). Findings by immunofluorescence showed that PAK4 was expressed in both nucleus and cytoplasm, and there was a reduction in group shPAK4 in comparison to group shControl and Control, which also verified a successful construction of stable shPAK4 cells (Figure 1C,1D) as well as western blot (Figure 1E). Both results indicated PAK4 was down-regulated in group shPAK4 in comparison to group shControl and Control. Consistent with preceding outcomes, the stable shPAK4 cells showed an attenuated proliferation by MTT or CCK8 assays in comparison to group shControl and Control (P<0.05 or 0.01; Figure 1F,1G).
HepG2 cells are arrested at G2/M phase following knockdown of PAK4
Since the inhibition in proliferation is usually associated with cell cycle arrest, we wonder whether the knockdown of PAK4 results in cell cycle arrest in HepG2 cells. The data by FCM showed that cells exhibited an alteration in cell cycle distribution in group shPAK4, and the proportion of cells was up-regulated at hour 72 and 96 during G2/M phase (Figure 2A,2B), indicating knockdown PAK4 induced arrest at G2/M phase.
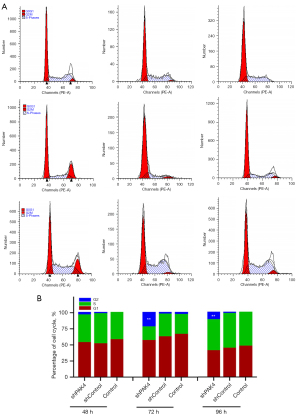
Knockdown PAK4 triggers autophagy phenotype in HepG2 cells
To investigate whether knockdown of PAK4 induces autophagy, autophagosome was checked by MDC staining in HepG2 cells. As results show, compared with shControl group, there was a larger proportion of fluorescence-positive autophagic vacuoles in group shPAK4 (P<0.01; Figure 3A,3B). For further confirmation, western blot was conducted to detect the conversion of LC3-I to LC3-II. Consistent with MDC assay, the expression ratio of LC3-II/LC3-I increased for group shPAK4 (P<0.01; Figure 3C,3D). To sum up, there was a negative correlation between PAK4’s expression and cell autophagy in HepG2.
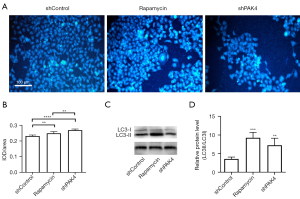
Knockdown PAK4 up-regulates expression in p53 and blockades mTOR/p-AKT signaling
p53 is a pivotal regulator in cell cycle. We detected its expression using immunofluorescence and western blot in PAK4-knockdown HepG2 cells. The findings showed there was a statistically significant up-regulation of p53 in group shPAK4 compared with group shControl and Control (Figure 4A-4C), implying that PAK4 negatively regulated p53 in protein. In addition, both mTOR and p-AKT/AKT manifested decreased expressions (Figure 4D-4H). It suggested that high expression of p53 with blocked mTOR/p-AKT signaling in PAK4-knockdown cells.
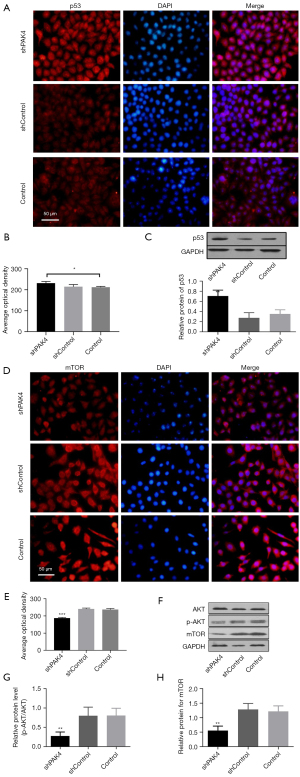
Discussion
Although p53 is traditionally considered a checkpoint molecule to G1 phase, researches have increasingly revealed that activation of p53 leads to cell cycle arrest at G1/S and G2/M phases (11-13,29). p53-p21 pathway represses cell cycle genes, most of which are essential regulators of G2 phase and mitosis (13). And then, we detected decreased proliferation and cell cycle arrest at G2/M with high expression in p53 in the PAK4-knockdown cells, a phenomenon indicating that the knockdown-induced arrest might be mediated by p53 during G2/M phase in the present study.
Research is rather limited when it comes to the relationship between PAK4 and p53. Although there are reports indicating that PAK4 is upstream to p53, the hierarchy of molecular events is still nubilous during the signal processes connecting PAK4 and p53 (30,31). However, we analyzed that PAK4 has a negative regulation to p53 because of their opposite expressions in the study, supported by the inaction of p53 due to its phosphorylation by PAK4 (14). Similarly, a study shows that PAK4 enhances binding between p53 and murine double minute 2, which promotes ubiquitination-mediated p53 degradation and increases activity of glucose-6-phosphate dehydrogenase on pentose phosphate pathway, resulting in an increased multiplication of cells in colon cancer (32).
It is well-known that impaired cell proliferation results from not only cell cycle arrest but enhanced death. Since the death was not from apoptosis in PAK4-knockdown hepatocarcinoma cells in our previous study (33), and p53 (25,26,34) and PAKs (16,19,21) were relevant with autophagy in cancer cells, the present study focused on autophagy. To date, main functional forms in autophagy are proposed to be cytotoxic, cytostatic, and cytoprotective in the context of antitumor therapy: cytotoxic autophagy leads to cancer cell death, cytostatic autophagy inhibits cancer cell propagation, and cytoprotective autophagy enhances cancer cells’ drug sensitivity (35). Our findings showed an increased autophagosome by MDC staining and expression of autophagic biomarker LC3-II/LC3-I, suggesting an increased autophagy inhibiting propagation in PAK4-knockdown HepG2 cells.
Some studies prove that p53-activated cell autophagy, as well as PAKs in tumors (20,21), are regulated via AKT and/or mTOR (25-28,34). Also, mTOR/AKT displays a close relationship with cell autophagy. The inhibition of mTOR/AKT signaling enhances autophagy via facilitating conversion of LC3-I to LC3-II in vascular smooth muscle cells (36). Meanwhile, autophagy is induced along with an inactivation in mTOR/AKT pathway in human breast cancer cells and cervical cancer cells (37,38), while high expression of AKT suppresses autophagy in ovarian cancer (39). In addition, mTOR/AKT is known as a canonical intracellular oncogenic pathway in regulation of tumor cell proliferation (40). Among multiple types of carcinoma, inhibition of mTOR/AKT could enable cell arrests at G2/M phase (41-43). Comparably, our findings exhibited low expressions in mTOR and mTOR’s target, p-AKT, suggesting the signaling was associated with the cellular events of cell cycle and autophagy, in PAK4-knockdown HepG2 cells.
Conclusions
The reduced proliferation in PAK4-knockdown HepG2 cells is caused by cell autophagy, mediated by activated p53 and damp mTOR/p-AKT signaling.
Acknowledgments
Funding: The study was supported by
Footnote
Reporting Checklist: The authors have completed the MDAR reporting checklist. Available at https://tcr.amegroups.com/article/view/10.21037/tcr-22-2272/rc
Data Sharing Statement: Available at https://tcr.amegroups.com/article/view/10.21037/tcr-22-2272/dss
Peer Review File: Available at https://tcr.amegroups.com/article/view/10.21037/tcr-22-2272/prf
Conflicts of Interest: All authors have completed the ICMJE uniform disclosure form (available at https://tcr.amegroups.com/article/view/10.21037/tcr-22-2272/coif). The authors have no conflicts of interest to declare.
Ethical Statement: The authors are accountable for all aspects of the work in ensuring that questions related to the accuracy or integrity of any part of the work are appropriately investigated and resolved. The study was conducted in accordance with the Declaration of Helsinki (as revised in 2013). Institutional ethical approval and informed consent were waived.
Open Access Statement: This is an Open Access article distributed in accordance with the Creative Commons Attribution-NonCommercial-NoDerivs 4.0 International License (CC BY-NC-ND 4.0), which permits the non-commercial replication and distribution of the article with the strict proviso that no changes or edits are made and the original work is properly cited (including links to both the formal publication through the relevant DOI and the license). See: https://creativecommons.org/licenses/by-nc-nd/4.0/.
References
- Shu XR, Wu J, Sun H, et al. PAK4 confers the malignance of cervical cancers and contributes to the cisplatin-resistance in cervical cancer cells via PI3K/AKT pathway. Diagn Pathol 2015;10:177. [Crossref] [PubMed]
- Santiago-Gómez A, Kedward T, Simões BM, et al. PAK4 regulates stemness and progression in endocrine resistant ER-positive metastatic breast cancer. Cancer Lett 2019;458:66-75. [Crossref] [PubMed]
- Tyagi N, Marimuthu S, Bhardwaj A, et al. p-21 activated kinase 4 (PAK4) maintains stem cell-like phenotypes in pancreatic cancer cells through activation of STAT3 signaling. Cancer Lett 2016;370:260-7. [Crossref] [PubMed]
- Callegari E, D'Abundo L, Guerriero P, et al. miR-199a-3p Modulates MTOR and PAK4 Pathways and Inhibits Tumor Growth in a Hepatocellular Carcinoma Transgenic Mouse Model. Mol Ther Nucleic Acids 2018;11:485-93. [Crossref] [PubMed]
- Hao C, Huang W, Li X, et al. Development of 2, 4-diaminoquinazoline derivatives as potent PAK4 inhibitors by the core refinement strategy. Eur J Med Chem 2017;131:1-13. [Crossref] [PubMed]
- Abu Aboud O, Chen CH, Senapedis W, et al. Dual and Specific Inhibition of NAMPT and PAK4 By KPT-9274 Decreases Kidney Cancer Growth. Mol Cancer Ther 2016;15:2119-29. [Crossref] [PubMed]
- Kaufmann KB, Garcia-Prat L, Liu Q, et al. A stemness screen reveals C3orf54/INKA1 as a promoter of human leukemia stem cell latency. Blood 2019;133:2198-211. [Crossref] [PubMed]
- Bompard G, Rabeharivelo G, Frank M, et al. Subgroup II PAK-mediated phosphorylation regulates Ran activity during mitosis. J Cell Biol 2010;190:807-22. [Crossref] [PubMed]
- Bompard G, Rabeharivelo G, Cau J, et al. P21-activated kinase 4 (PAK4) is required for metaphase spindle positioning and anchoring. Oncogene 2013;32:910-9. [Crossref] [PubMed]
- Kim EM, Jung CH, Kim J, et al. The p53/p21 Complex Regulates Cancer Cell Invasion and Apoptosis by Targeting Bcl-2 Family Proteins. Cancer Res 2017;77:3092-100. [Crossref] [PubMed]
- Schade AE, Fischer M, DeCaprio JA RB. p130 and p107 differentially repress G1/S and G2/M genes after p53 activation. Nucleic Acids Res 2019;47:11197-208. [Crossref] [PubMed]
- Liu X, Liu J. Tanshinone I induces cell apoptosis by reactive oxygen species-mediated endoplasmic reticulum stress and by suppressing p53/DRAM-mediated autophagy in human hepatocellular carcinoma. Artif Cells Nanomed Biotechnol 2020;48:488-97. [Crossref] [PubMed]
- Engeland K. Cell cycle arrest through indirect transcriptional repression by p53: I have a DREAM. Cell Death Differ 2018;25:114-32. [Crossref] [PubMed]
- Xu HT, Lai WL, Liu HF, et al. PAK4 Phosphorylates p53 at Serine 215 to Promote Liver Cancer Metastasis. Cancer Res 2016;76:5732-42. [Crossref] [PubMed]
- Aksnes H, Ree R, Arnesen T. Co-translational, Post-translational, and Non-catalytic Roles of N-Terminal Acetyltransferases. Mol Cell 2019;73:1097-114. [Crossref] [PubMed]
- Mercado-Pimentel ME, Miller C, Rolph DN, et al. Inhibiting p21-Activated Kinase Induces Cell Death in Vestibular Schwannoma and Meningioma via Mitotic Catastrophe. Otol Neurotol 2017;38:139-46. [Crossref] [PubMed]
- Kim JH, Seo Y, Jo M, et al. Interrogation of kinase genetic interactions provides a global view of PAK1-mediated signal transduction pathways. J Biol Chem 2020;295:16906-19. [Crossref] [PubMed]
- Zhu S, Tang X, Gao X, et al. hsa_circ_0013401 Accelerates the Growth and Metastasis and Prevents Apoptosis and Autophagy of Neuroblastoma Cells by Sponging miR-195 to Release PAK2. Oxid Med Cell Longev 2021;2021:9936154. [Crossref] [PubMed]
- Jayashankar V, Finicle BT, Edinger AL. Starving PTEN-deficient prostate cancer cells thrive under nutrient stress by scavenging corpses for their supper. Mol Cell Oncol 2018;5:e1472060. [Crossref] [PubMed]
- Wang Z, Jia G, Li Y, et al. Clinicopathological signature of p21-activated kinase 1 in prostate cancer and its regulation of proliferation and autophagy via the mTOR signaling pathway. Oncotarget 2017;8:22563-80. [Crossref] [PubMed]
- Dou Q, Chen HN, Wang K, et al. Ivermectin Induces Cytostatic Autophagy by Blocking the PAK1/Akt Axis in Breast Cancer. Cancer Res 2016;76:4457-69. [Crossref] [PubMed]
- Bu H, Tan S, Yuan B, et al. Therapeutic potential of IBP as an autophagy inducer for treating lung cancer via blocking PAK1/Akt/mTOR signaling. Mol Ther Oncolytics 2020;20:82-93. [Crossref] [PubMed]
- Römermann D, Ansari N, Schultz-Moreira AR, et al. Absence of Atg7 in the liver disturbed hepatic regeneration after liver injury. Liver Int 2020;40:1225-38. [Crossref] [PubMed]
- Gupta R, Ambasta RK. Pravir Kumar. Autophagy and apoptosis cascade: which is more prominent in neuronal death? Cell Mol Life Sci 2021;78:8001-47. [Crossref] [PubMed]
- Bernard M, Yang B, Migneault F, et al. Autophagy drives fibroblast senescence through MTORC2 regulation. Autophagy 2020;16:2004-16. [Crossref] [PubMed]
- Akeno N, Miller AL, Ma X, et al. p53 suppresses carcinoma progression by inhibiting mTOR pathway activation. Oncogene 2015;34:589-99. [Crossref] [PubMed]
- Hu L, Wang Y, Chen Z, et al. Hsp90 Inhibitor SNX-2112 Enhances TRAIL-Induced Apoptosis of Human Cervical Cancer Cells via the ROS-Mediated JNK-p53-Autophagy-DR5 Pathway. Oxid Med Cell Longev 2019;2019:9675450. [Crossref] [PubMed]
- Magalhães-Novais S, Bermejo-Millo JC, Loureiro R, et al. Cell quality control mechanisms maintain stemness and differentiation potential of P19 embryonic carcinoma cells. Autophagy 2020;16:313-33. [Crossref] [PubMed]
- Fischer M, Quaas M, Steiner L, et al. The p53-p21-DREAM-CDE/CHR pathway regulates G2/M cell cycle genes. Nucleic Acids Res 2016;44:164-74. [Crossref] [PubMed]
- Murray BW, Guo C, Piraino J, et al. Small-molecule p21-activated kinase inhibitor PF-3758309 is a potent inhibitor of oncogenic signaling and tumor growth. Proc Natl Acad Sci U S A 2010;107:9446-51. [Crossref] [PubMed]
- Liu X, Min S, Wu N, et al. miR-193a-3p inhibition of the Slug activator PAK4 suppresses non-small cell lung cancer aggressiveness via the p53/Slug/L1CAM pathway. Cancer Lett 2019;447:56-65. [Crossref] [PubMed]
- Zhang X, Zhang X, Li Y, et al. PAK4 regulates G6PD activity by p53 degradation involving colon cancer cell growth. Cell Death Dis 2017;8:e2820. [Crossref] [PubMed]
- Li Q, Zhang X, Wei N, et al. p21-activated kinase 4 as a switch between caspase-8 apoptosis and NF-κB survival signals in response to TNF-α in hepatocarcinoma cells. Biochem Biophys Res Commun 2018;503:3003-10. [Crossref] [PubMed]
- Feng Z, Levine AJ. The regulation of energy metabolism and the IGF-1/mTOR pathways by the p53 protein. Trends Cell Biol 2010;20:427-34. [Crossref] [PubMed]
- Finnegan RM, Elshazly AM, Schoenlein PV, et al. Therapeutic Potential for Targeting Autophagy in ER+ Breast Cancer. Cancers (Basel) 2022;14:4289. [Crossref] [PubMed]
- Sung JY, Lee KY, Kim JR, et al. Interaction between mTOR pathway inhibition and autophagy induction attenuates adriamycin-induced vascular smooth muscle cell senescence through decreased expressions of p53/p21/p16. Exp Gerontol 2018;109:51-8. [Crossref] [PubMed]
- Xue L, Zhang WJ, Fan QX, et al. Licochalcone A inhibits PI3K/Akt/mTOR signaling pathway activation and promotes autophagy in breast cancer cells. Oncol Lett 2018;15:1869-73. [PubMed]
- Tsai JP, Lee CH, Ying TH, et al. Licochalcone A induces autophagy through PI3K/Akt/mTOR inactivation and autophagy suppression enhances Licochalcone A-induced apoptosis of human cervical cancer cells. Oncotarget 2015;6:28851-66. [Crossref] [PubMed]
- Luan W, Pang Y, Li R, et al. Akt/mTOR-Mediated Autophagy Confers Resistance To BET Inhibitor JQ1 In Ovarian Cancer. Onco Targets Ther 2019;12:8063-74. [Crossref] [PubMed]
- Alzahrani AS. PI3K/Akt/mTOR inhibitors in cancer: At the bench and bedside. Semin Cancer Biol 2019;59:125-32. [Crossref] [PubMed]
- Zhang XH, Wang XY, Zhou ZW, et al. The combination of digoxin and GSK2606414 exerts synergistic anticancer activity against leukemia in vitro and in vivo. Biofactors 2017;43:812-20. [Crossref] [PubMed]
- Yu CC, Huang HB, Hung SK, et al. AZD2014 Radiosensitizes Oral Squamous Cell Carcinoma by Inhibiting AKT/mTOR Axis and Inducing G1/G2/M Cell Cycle Arrest. PLoS One 2016;11:e0151942. [Crossref] [PubMed]
- Wu T, Dong X, Yu D, et al. Natural product pectolinarigenin inhibits proliferation, induces apoptosis, and causes G2/M phase arrest of HCC via PI3K/AKT/mTOR/ERK signaling pathway. Onco Targets Ther 2018;11:8633-42. [Crossref] [PubMed]