Beginning of clinical treatment using the 1.5 Tesla MR-Linac system in Japan: a narrative review
Introduction
In radiation therapy, the technique for verification of the patient’s position has been gradually improving (1). In current image-guided radiotherapy (IGRT), bone images of the patient are used for position verification in many institutions. Bone images are obtained by using an electronic portal imaging device (EPID) while the patient is lying on the radiation therapy table to verify the patient’s position. Leong reported the method and it has been used since the 1990s (2). With the progress of research, images of soft tissue began to be used instead of bone images for position verification. Although it does not provide the same soft tissue resolution as that of diagnostic computed tomography (CT), position verification using cone-beam CT (CBCT) was initiated (3). Such advances in IGRT became the foundation for the development of highly precise radiation therapies such as stereotactic body radiation therapy (SBRT) and intensity-modulated radiation therapy (IMRT). Research on magnetic resonance imaging (MRI)-based IGRT techniques was carried out to obtain better soft tissue contrast (4). A device for radiation therapy was devised by combining a linear accelerator and a 1.5 Tesla MRI system (5). This radiation therapy device that enables IGRT and online adaptive radiotherapy (ART) using 1.5 Tesla MRI is called 1.5 Tesla magnetic resonance linear accelerator (MR-Linac). In Europe, the first patient received treatment as part of a study using the 1.5 Tesla MR-Linac in 2017 (6). 1.5 Tesla MR-Linac obtained CE-mark certification in August 2018 and clinical treatment using the device has been conducted in Europe. In Japan, clinical treatment using the device started in 2021. The use of 1.5 Tesla MR-Linac for radiation therapy has gained attention and is expected to make significant contributions in the future. In this review, we provide an overview and discuss future prospects of 1.5 Tesla MR-Linac. We present this article in accordance with the Narrative Review reporting checklist (available at https://tcr.amegroups.com/article/view/10.21037/tcr-23-1649/rc).
Methods
A search was conducted in PubMed for articles related to 1.5 Tesla MR-Linac and MR-Linac. Clinical treatment with 1.5 Tesla MR-Linac has been conducted in our institution since February 2022. N.T. searched the literature and selected reports that are important for understanding an overview of 1.5 Tesla MR-Linac (Table 1).
Table 1
Items | Specification |
---|---|
Date of search | March 1st, 2023 |
Databases and other sources searched | PubMed |
Search terms used | 1.5 T MR-Linac or MR-Linac |
Timeframe | Until November 2023 |
Inclusion criteria | Original article or Review article; only English |
Selection process | N.T. read the titles and abstracts, and also read the text of any documents that seemed related. N.T. selected the documents that are important for understanding an overview of 1.5 Tesla MR-Linac |
MR-Linac, magnetic resonance linear accelerator.
SBRT by using 1.5 Tesla MR-Linac has so far performed for more than 200 patients in our institution (Figure 1). An overview of 1.5 Tesla MR-Linac based on the literatures and our experience is provided here.
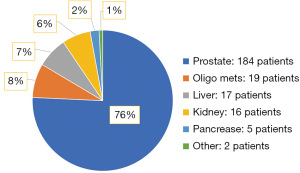
Effects of magnetic fields on radiation dose
X-rays themselves are not affected by a magnetic field. However, the trajectory of the generated electrons changes due to the Lorentz force (7). When examining the influence of a magnetic field on percentage depth dose, it is observed that in the presence of a magnetic field, the maximum depth becomes shallower and the dose in deeper regions tends to be lower (8). At the boundary of substances with different electron densities, a phenomenon called the electron return effect occurs. This is a phenomenon in which electrons that have exited the body in the air return to the surface of the body due to the Lorentz force (7). There is another phenomenon called the electron streaming effect that occurs when X-rays enter or exit an object (9). In this phenomenon, generated electrons tend to move in the direction of a magnetic field, and in the human body, this phenomenon can cause electrons to hit areas such as the jaw (10). In the radiation treatment planning system of a 1.5 Tesla MR-Linac, the dose distribution map is created with the influence of these magnetic fields taken into account.
IGRT using 1.5 Tesla MRI
The 1.5 Tesla MR-Linac has three strengths compared to a conventional Linac. The first is IGRT using 1.5 Tesla MRI. In recent years, position verification using CBCT has been used instead of position verification using EPID (3). While X-rays have been used for positional alignment based on bones, even if the positions of the bones are used for alignment, there can still be some discrepancies with organs and tumors within the body. In CBCT, images are acquired by using kilovolt X-rays, but image quality is inferior to that in diagnostic CT scans. Noel et al. reported that even a low-field MR-Linac provided better anatomic visualization than that provided by CBCT (11). CBCT has the advantage of easy attachment of the system to a Linac, but it has the disadvantage of having slightly poor soft-tissue contrast, although it provides some understanding of internal organs. In contrast, the 1.5 Tesla MR-Linac allows positional verification using 1.5 Tesla MRI (Figure 2). The same imaging equipment as that used for diagnostic MRI scanners in used for 1.5 Tesla MRI (6). Using an MRI scanner with the same specifications as the diagnostic imaging equipment enables not only positional alignment but also the acquisition of functional images. The application of functional images to radiation therapy holds promising potential for the future.
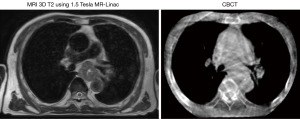
By utilizing 1.5 Tesla MRI, images with soft tissue resolution that is superior to that of images obtained by conventional CBCT can be used for radiation therapy in the mediastinal, abdominal and pelvic regions, and thus the accuracy of position verification is improved compared to that of CBCT-based positional verification.
MR-guided online ART
The second strength of 1.5 Tesla MR-Linac is that it has a simple workflow for administering online ART. ART is a method for re-planning during the course of radiotherapy (12). On the other hand, online ART is a method for re-planning while the patient is on the treatment table. Online ART is already being used for various types of radiotherapy such as brachytherapy. Low dose rate brachytherapy for patients with prostate cancer is online ART using ultrasound images and high dose rate brachytherapy for patients with cervical cancer is online ART using CT or MRI (13). In conventional external beam radiotherapy, when the shape of the tumor is changed, re-planning is generally performed and sometimes more than once. However, when re-planning is performed, it takes several days for the patient to receive radiotherapy with the latest plan. During that time, irradiation based on the initial plan will be performed. On the other hand, when 1.5 Tesla MR-Linac is used, ART is performed while the patients lying on the Linac treatment table. Therefore, it is possible to perform radiotherapy with a plan that is adapted to the shape of the tumor and the shapes of organ at risk (OAR) on that day (14). Daily ART using CBCT is also being considered, but, as mentioned above, CBCT has the disadvantage of being inferior to MRI in terms of soft tissue contrast (15).
By using 1.5 Tesla MR-Linac, it is possible to treat patients with daily ART based on 1.5 Tesla high-field MRI images (Figure 3).
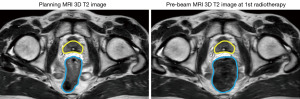
Motion monitoring
The third strength of 1.5 Tesla MR-Linac is that motion monitoring of inside the patient’s body can be performed during on-beam of treatment X-ray without extra radiation exposure. In order to confirm tumor motion when radiotherapy is being carried out using a conventional Linac, it is possible to confirm the movement of the tumor by observing the movement of a fiducial marker using X-ray fluoroscopy instead of observing the movement of the tumor itself (16). Fiducial markers are often used to improve the accuracy of radiation therapy. However, when a fiducial marker is placed, pain, bleeding and, depending on the site, pneumothorax and organ puncture may occur (17). On the other hand, by using 1.5 Tesla MR-Linac, it is possible to see the movement of the tumor itself without radiation exposure and the use of a fiducial marker.
By using 1.5 Tesla MRI cine images, the tumor location can be monitored in real time during radiotherapy, thus further increasing the accuracy of radiotherapy without the use of a fiducial marker (Video 1).
Treatment workflow of 1.5 Tesla MR-Linac
The workflow of treatment with 1.5 Tesla MR-Linac first involved obtaining radiotherapy planning images in the same way as that for conventional radiotherapy planning (Figure 4). Planning MRI is not mandatory for radiotherapy planning, and CT alone can be used. As in the case of conventional radiotherapy, segmentations of OAR and the tumor are performed on the planning images to create a dose distribution. The average electron densities of OAR, the tumor and the patient’s body are obtained from CT images. These electron densities are used for plan adaptation on MRI of daily radiotherapy because the electron density is not known in MRI. By assigning these electron densities to segmentation on MRI, plan adaptation can be performed for MRI (18).
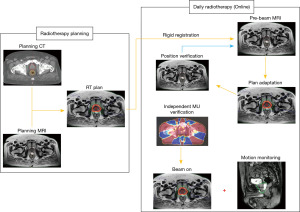
On the day of radiotherapy, rigid registration using the planning image and pre-beam MRI on that day is performed. After rigid registration, plan adaptation is performed. There are two methods for plan adaptation: adapt to position (ATP) workflow and adapt to shape (ATS) workflow (12). ATP is a method in which the plan is adapted by considering only the misalignment of the tumor in the planning image and pre-beam MRI. On the other hand, ATS is a method of performing re-segmentation of OAR and the tumor in the pre-beam MRI of the day and adapting the plan. ATS is a method of adapting the plan by considering not only positional displacement but also changes in the shapes of OAR and the tumor. After plan adaptation is completed, MRI for position verification is performed to check whether there are any changes in the tumor position during adaptation. If there is a change in the position of the tumor, plan adaptation is performed for the position verification MRI again to correct it. If there is no positional deviation of the tumor in position verification MRI or the deviation is within an acceptable range, an independent MU verification will be performed and irradiation will be started. Motion monitoring during irradiation is performed using MRI cine images. After irradiation, MRI may be performed to check for any positional deviation, but this is optional.
Advantage of MR-Linac
As mentioned above, the three advantages of 1.5 Tesla MR-Linac are position verification with 1.5 Tesla MRI, a workflow that allows easy online ART, and motion monitoring during irradiation without the use of a marker. It is expected that these advantages will improve the accuracy of radiation therapy and reduce side effects. Henke et al. showed that unnecessary dose constraints for OAR are reduced and planning target volume (PTV) coverage is increased by online ART using MR-Linac compared with those when using conventional CBCT-based Linac (19). Nierer et al. showed that MR-guided online ART improves dosimetric parameters for patients with liver, lung, abdominal lymph nodes, pancreas and prostate cancer (20). There is a report showing not only improvements in dosimetric parameters but also reduction in acute injury by using MR-Linac. Kishan et al. showed that patients who received radiotherapy by MR-Linac had fewer side effects than those in patients who received conventional CBCT radiation therapy (21). They treated patients with prostate cancer by SBRT. In their study, the MR-Linac group was treated with a PTV margin of 2 mm and the CBCT group was treated with a PTV margin of 4 mm. The incidence of grade 2 or higher acute genitourinary toxicity was significantly lower in the MRI group than in the CBCT group.
1.5 Tesla MR-Linac in Japan and future prospects of 1.5 Tesla MR-Linac
There are currently three institutions in Japan in which 1.5 Tesla MR-Linac has been installed (22,23). In Japan, treatment using 1.5 Tesla MR-Linac started in December 2021 (22). Much interest has been shown in 1.5 Tesla MR-Linac not only for clinical use but also as for research. Facilities with MR-Linac in Japan have formed a team and are proceeding with clinical studies and research (14).
By using 1.5 Tesla MR-Linac, it is possible to reduce the PTV margin compared to that using conventional radiation therapy equipment. Since side effects can be expected to be reduced compared to those in the past, it will also be possible to try to increase the dose of radiation therapy. In the future, it will be possible to increase the radiation dose per fraction and perform SBRT in a smaller number of fractions (24).
1.5 Tesla MR-Linac treatment can be considered as a treatment method that is still in the process of development. By using a 1.5 Tesla MRI, it is possible to obtain images that are equivalent to those obtained by diagnostic MRI. Which MRI sequence is suitable for treatment of which area is an issue for which further study is need. In addition, since functional images such as images obtained by diffusion-weighted imaging can be acquired, precision medicine such as radiation dose adjustment by such images is also an issue for further study. A consortium has been formed to advance research on 1.5 T MR-Linac (25). There are several tumor site groups. They are discussing the research and clinical applications of 1.5 Tesla MR-Linac in each organ, and it is expected that treatment using 1.5 Tesla MR-Linac will continue to advance.
Problems with and limitations of MR-Linac
There are some problems to use 1.5 Tesla MR-Linac. First, the delivery of each fraction of radiation therapy takes longer when using 1.5 Tesla MR-Linac than when using a conventional Linac. Lakomy et al. reported that the median duration of radiotherapy with the ATP workflow was 30 minutes (range, 17–66 minutes) and that of radiotherapy with the ATS workflow was 42 minutes (range, 30–65 minutes) (26). Either ATP or ATS workflows need plan adaptation, which increases total radiotherapy time.
The second problem is staff training. The use of 1.5 Tesla MR-Linac is a new treatment situation in which patients are irradiated in a magnetic field. Radiotherapy technologists (RTTs), radiation oncologists and nurses must be fully aware of the danger of staying in magnetic fields. And, RTTs, medical physicists and radiation oncologists need to be familiar with the online ART workflow. ART with MR-Linac will take a long time unless all of the staff members are familiar with it. Radiation oncologists must be fully aware of the tumor and OAR for radiotherapy that a patient will receive, especially since re-segmentation is very time-consuming. Recently published study have been allowed technicians to re-segment OAR and the tumor in online ART of MR-Linac (27). In any case, staff education is important for smooth treatment by online ART.
The third problem is that it is difficult to treat patients for whom MRI cannot be performed. For example, MRI cannot be performed for patients with pacemakers, cochlear implants, metal implants of unknown material and claustrophobia. Even if an MRI-compatible metal is implanted, it may not be suitable if it is located near the lesion and may disturb the image.
Conclusions
Clinical treatment using 1.5 Tesla MR-Linac has begun in Japan. There are some precautions to be taken when using it. However, there are advantages such as IGRT using 1.5 Tesla MRI, MR-guided online ART, and motion monitoring during radiotherapy beam on. By using 1.5 Tesla MR-Linac, we can provide patients with higher precision radiotherapy.
Acknowledgments
Funding: None.
Footnote
Reporting Checklist: The authors have completed the Narrative Review reporting checklist. Available at https://tcr.amegroups.com/article/view/10.21037/tcr-23-1649/rc
Peer Review File: Available at https://tcr.amegroups.com/article/view/10.21037/tcr-23-1649/prf
Conflicts of Interest: All authors have completed the ICMJE uniform disclosure form (available at https://tcr.amegroups.com/article/view/10.21037/tcr-23-1649/coif). N.T. serves as an unpaid editorial board member of Translational Cancer Research from February 2022 to January 2024. K.J. reports grants from Elekta, K.K. The other authors have no conflicts of interest to declare.
Ethical Statement: The authors are accountable for all aspects of the work in ensuring that questions related to the accuracy or integrity of any part of the work are appropriately investigated and resolved. All patients give consent and signed a consent form of Department of Radiation Oncology, Tohoku University Graduate School of Medicine in Japanese.
Open Access Statement: This is an Open Access article distributed in accordance with the Creative Commons Attribution-NonCommercial-NoDerivs 4.0 International License (CC BY-NC-ND 4.0), which permits the non-commercial replication and distribution of the article with the strict proviso that no changes or edits are made and the original work is properly cited (including links to both the formal publication through the relevant DOI and the license). See: https://creativecommons.org/licenses/by-nc-nd/4.0/.
References
- Verellen D, De Ridder M, Storme G A. (short) history of image-guided radiotherapy. Radiother Oncol 2008;86:4-13. [Crossref] [PubMed]
- Leong J. Use of digital fluoroscopy as an on-line verification device in radiation therapy. Phys Med Biol 1986;31:985-92. [Crossref] [PubMed]
- Jaffray DA, Siewerdsen JH, Wong JW, et al. Flat-panel cone-beam computed tomography for image-guided radiation therapy. Int J Radiat Oncol Biol Phys 2002;53:1337-49. [Crossref] [PubMed]
- Raaymakers BW, Raaijmakers AJ, Kotte AN, et al. Integrating a MRI scanner with a 6 MV radiotherapy accelerator: dose deposition in a transverse magnetic field. Phys Med Biol 2004;49:4109-18. [Crossref] [PubMed]
- Raaymakers BW, Lagendijk JJ, Overweg J, et al. Integrating a 1.5 T MRI scanner with a 6 MV accelerator: proof of concept. Phys Med Biol 2009;54:N229-37.
- Raaymakers BW, Jürgenliemk-Schulz IM, Bol GH, et al. First patients treated with a 1.5 T MRI-Linac: clinical proof of concept of a high-precision, high-field MRI guided radiotherapy treatment. Phys Med Biol 2017;62:L41-50. [Crossref] [PubMed]
- Raaijmakers AJ, Raaymakers BW, Lagendijk JJ. Magnetic-field-induced dose effects in MR-guided radiotherapy systems: dependence on the magnetic field strength. Phys Med Biol 2008;53:909-23. [Crossref] [PubMed]
- O’Brien DJ, Dolan J, Pencea S, et al. Relative dosimetry with an MR-linac: Response of ion chambers, diamond, and diode detectors for off-axis, depth dose, and output factor measurements. Med Phys 2018;45:884-97. [Crossref] [PubMed]
- Malkov VN, Hackett SL, Wolthaus JWH, et al. Monte Carlo simulations of out-of-field surface doses due to the electron streaming effect in orthogonal magnetic fields. Phys Med Biol 2019;64:115029. [Crossref] [PubMed]
- Liu H, Ding S, Wang B, et al. In-Air Electron Streaming Effect for Esophageal Cancer Radiotherapy With a 1.5 T Perpendicular Magnetic Field: A Treatment Planning Study. Front Oncol 2020;10:607061. [Crossref] [PubMed]
- Noel CE, Parikh PJ, Spencer CR, et al. Comparison of onboard low-field magnetic resonance imaging versus onboard computed tomography for anatomy visualization in radiotherapy. Acta Oncol 2015;54:1474-82. [Crossref] [PubMed]
- Winkel D, Bol GH, Kroon PS, et al. Adaptive radiotherapy: The Elekta Unity MR-linac concept. Clin Transl Radiat Oncol 2019;18:54-9. [Crossref] [PubMed]
- Tan Mbbs Mrcp Frcr Md LT. Image-guided Adaptive Radiotherapy in Cervical Cancer. Semin Radiat Oncol 2019;29:284-98. [Crossref] [PubMed]
- Okamoto H, Igaki H, Chiba T, et al. Practical guidelines of online MR-guided adaptive radiotherapy. J Radiat Res 2022;63:730-40. [Crossref] [PubMed]
- Branco D, Mayadev J, Moore K, et al. Dosimetric and feasibility evaluation of a CBCT-based daily adaptive radiotherapy protocol for locally advanced cervical cancer. J Appl Clin Med Phys 2023;24:e13783. [Crossref] [PubMed]
- Shiinoki T, Hanazawa H, Yuasa Y, et al. Verification of respiratory-gated radiotherapy with new real-time tumour-tracking radiotherapy system using cine EPID images and a log file. Phys Med Biol 2017;62:1585-99. [Crossref] [PubMed]
- Trumm CG, Häussler SM, Muacevic A, et al. CT fluoroscopy-guided percutaneous fiducial marker placement for CyberKnife stereotactic radiosurgery: technical results and complications in 222 consecutive procedures. J Vasc Interv Radiol 2014;25:760-8. [Crossref] [PubMed]
- Wang C, Chao M, Lee L, et al. MRI-based treatment planning with electron density information mapped from CT images: a preliminary study. Technol Cancer Res Treat 2008;7:341-8. [Crossref] [PubMed]
- Henke L, Kashani R, Yang D, et al. Simulated Online Adaptive Magnetic Resonance-Guided Stereotactic Body Radiation Therapy for the Treatment of Oligometastatic Disease of the Abdomen and Central Thorax: Characterization of Potential Advantages. Int J Radiat Oncol Biol Phys 2016;96:1078-86. [Crossref] [PubMed]
- Nierer L, Eze C, da Silva Mendes V, et al. Dosimetric benefit of MR-guided online adaptive radiotherapy in different tumor entities: liver, lung, abdominal lymph nodes, pancreas and prostate. Radiat Oncol 2022;17:53. [Crossref] [PubMed]
- Kishan AU, Ma TM, Lamb JM, et al. Magnetic Resonance Imaging-Guided vs Computed Tomography-Guided Stereotactic Body Radiotherapy for Prostate Cancer: The MIRAGE Randomized Clinical Trial. JAMA Oncol 2023;9:365-73. [Crossref] [PubMed]
- Uno T, Tsuneda M, Abe K, et al. A new workflow of the on-line 1.5-T MR-guided adaptive radiation therapy. Jpn J Radiol 2023;41:1316-22. [Crossref] [PubMed]
- Tanaka S, Kadoya N, Ishizawa M, et al. Evaluation of Unity 1.5 T MR-linac plan quality in patients with prostate cancer. J Appl Clin Med Phys 2023;24:e14122. [Crossref] [PubMed]
- Mohajer J, Dunlop A, Mitchell A, et al. Feasibility of MR-guided ultrahypofractionated radiotherapy in 5, 2 or 1 fractions for prostate cancer. Clin Transl Radiat Oncol 2020;26:1-7. [Crossref] [PubMed]
- de Mol van Otterloo SR, Christodouleas JP, Blezer ELA, et al. The MOMENTUM Study: An International Registry for the Evidence-Based Introduction of MR-Guided Adaptive Therapy. Front Oncol 2020;10:1328. [Crossref] [PubMed]
- Lakomy DS, Yang J, Vedam S, et al. Clinical Implementation and Initial Experience With a 1.5 Tesla MR-Linac for MR-Guided Radiation Therapy for Gynecologic Cancer: An R-IDEAL Stage 1 and 2a First in Humans Feasibility Study of New Technology Implementation. Pract Radiat Oncol 2022;12:e296-305. [Crossref] [PubMed]
- Rasing MJA, Sikkes GG, Vissers NGPM, et al. Online adaptive MR-guided radiotherapy: Conformity of contour adaptation for prostate cancer, rectal cancer and lymph node oligometastases among radiation therapists and radiation oncologists. Tech Innov Patient Support Radiat Oncol 2022;23:33-40. [Crossref] [PubMed]