Drivers of neuroendocrine prostate cancer
Prostate cancer (PCa) is the most common malignancy in men and remains a leading cause of cancer death in males worldwide (1). The mainstay for patients with advanced and metastatic PCa, including castration-resistant disease, is hormonal therapy that targets the androgen receptor (AR) (androgen deprivation therapy) (2). Recently potent AR-targeted therapies were approved for the treatment of men with castration-resistant prostate cancer (CRPC), as de case of enzalutamide and abiraterone (3,4). While the use of these agents improves the survival of individuals with CRPC, most of them eventually develop resistance to therapy with a lethal outcome (5). This phenomenon may reflect an epithelial plasticity that enables tumor adaptation in response to AR-target therapies, which is not fully understood.
It has been highlighted that androgen-deprivation therapy frequently induces the emergence of highly aggressive prostate phenotypes with neuroendocrine features, also called neuroendocrine transdifferentiation (NEtD) (6). With the introduction of this new era of potent androgen receptor-targeted agents into the clinic, there is an evolving change in the clinical landscape of advanced PCa and treatment-related neuroendocrine prostate cancer (NEPC) is becoming an even more important condition to recognize.
NEPC is a high-risk, lethal subset of disease and is distinguished from prostate adenocarcinoma by the expression of neuroendocrine markers (as synaptophysin and chromogranin) and the loss of expression of the AR and PSA (6,7). Other neuroendocrine markers have been reported, although they are not typically used in the clinical practice (8). These markers include synaptic vesicle protein 2 (9), granin-A (10) and more recently the T-Box Brachyury (11) (Figure 1). Although some of these markers are promising, more studies are necessary before they can be used in clinical practice for detecting NEPC. NEPC is often referred to as representing only 2% of all diagnosed PCa (12). However, it is believed that probably occurs far more often, since the disease is not undistinguished of metastatic CRPC, and therefore, NEPC is underdiagnosed.
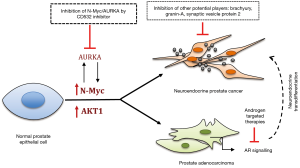
In 2010, Witte’s group reported the first functional study addressing the origin of PCa. The authors showed that basal cells from primary benign human prostate tissue can initiate PCa in mice through cooperative effects of AKT1 and ERG overexpression (13). Further studies from the group identified that prostate adenocarcinoma and squamous cell carcinoma can arise from a common basal cell precursor with deregulation of c-Myc expression and AKT1 (14). The proto-oncogene c-Myc is highly expressed in prostate adenocarcinomas, at variance of N-Myc that is only expressed in 5% of prostate adenocarcinomas, but is overexpressed and amplified in about 40% of NEPC (15), indicating a potential role of N-Myc as a critical oncoprotein in NEPC.
In a recent publication in Cancer Cell (16), Lee and colleagues (from Witte’s group) elegantly show the major role of N-Myc and AKT1 in NEPC phenotype and as potential targets for therapeutic intervention (Figure 1). The authors establish a novel in vivo model that reflect the histology and molecular features of human end-stage PCa with mixed NEPC and prostate adenocarcinoma, by deregulating the expression of N-Myc and AKT1 in primary human prostate epithelial cells. This outstanding model of NEPC (M-Myc/AKT1 tumors) showed to be androgen-independent, as demonstrated by the low or absent of AR expression, a similar feature of human CRPC and NEPCs (17). The importance of N-Myc in NEPC was highlighted by the contrasting results obtained by the combination of c-Myc and AKT1, which generates prostate adenosquamous carcinomas in the same system (16). These results also demonstrate the importance of different MYC members in the susceptibility to different kinds of cancer. Importantly, this work has brought a new in vivo tool for proper studies of NEPC, that lack of suitable models with good defined genetic drivers.
NEPC usually harbor genetic abnormalities also present in prostate adenocarcinoma as ETS rearrangements and PTEN mutations (17-19). The recently identified independent prognostic biomarker in prostate adenocarcinomas, Brachyury (20), showed also to be highly associated with tumors with neuroendocrine markers, which also present ETS overexpression and loss of PTEN (11). These studies indicate that NEPC type may arise from common clonal origin. Lee and colleagues (16) successful demonstrated that the NEPC and prostate adenocarcinoma can arise from a common prostate epithelial cell with N-Myc/myrAKT1 alteration, but not from luminal epithelial cells (where the benign neuroendocrine cells are present). Future studies will be necessary to clarify the influence of N-Myc/myrAKT1 in this subpopulation of cells.
Interestingly, the authors also showed that the incidence of NEPC tumors increases compared with adenocarcinoma when the N-Myc/myrAKT1 clones are propagated in castrate conditions (16), indicating that prostate adenocarcinomas undergo NEtD under selective pressure of androgen deprivation (Figure 1). Moreover, tumors with N-Myc overexpression presented stem cell-like properties that could act as repopulation clones of NEPC and consequently therapy resistance (16). These novel evidences are in agreement with a previous study where it was shown a correlation between acquisition of stem cell properties in PCa, NEtD and resistance to conventional chemotherapy (11) (Figure 1). It was been reported that patients with prostate adenocarcinoma that develop NEPC present amplification of both N-Myc and aurora A kinase (AURKA) (18). Based in these findings, Lee and colleagues (16), have shown that inhibition of AURKA and N-Myc (by positive feedback) by using the CD532 inhibitor, reduce tumor growth in vivo (Figure 1).
Concluding, the work published by Lee et al. in Cancer Cell (16) have provided novel and important data about the etiology and molecular basis of this aggressive subset of PCa, that could be further explored to increase our ability to diagnose NEPC at an earlier stage and may guide future pre-clinical studies for the treatment of patients with NEPC.
Acknowledgments
Funding: None.
Footnote
Provenance and Peer Review: This article was commissioned and reviewed by the Section Editor Peng Zhang (Department of Urology, Union Hospital, Tongji Medical College, Huazhong University of Science and Technology, Wuhan, China).
Conflicts of Interest: Both authors have completed the ICMJE uniform disclosure form (available at http://dx.doi.org/10.21037/tcr.2016.09.26). The authors have no conflicts of interest to declare.
Ethical Statement: The authors are accountable for all aspects of the work in ensuring that questions related to the accuracy or integrity of any part of the work are appropriately investigated and resolved.
Open Access Statement: This is an Open Access article distributed in accordance with the Creative Commons Attribution-NonCommercial-NoDerivs 4.0 International License (CC BY-NC-ND 4.0), which permits the non-commercial replication and distribution of the article with the strict proviso that no changes or edits are made and the original work is properly cited (including links to both the formal publication through the relevant DOI and the license). See: https://creativecommons.org/licenses/by-nc-nd/4.0/.
References
- Siegel RL, Miller KD, Jemal A. Cancer statistics, 2015. CA Cancer J Clin 2015;65:5-29. [Crossref] [PubMed]
- Chen CD, Welsbie DS, Tran C, et al. Molecular determinants of resistance to antiandrogen therapy. Nat Med 2004;10:33-9. [Crossref] [PubMed]
- Scher HI, Fizazi K, Saad F, et al. Increased survival with enzalutamide in prostate cancer after chemotherapy. N Engl J Med 2012;367:1187-97. [Crossref] [PubMed]
- de Bono JS, Logothetis CJ, Molina A, et al. Abiraterone and increased survival in metastatic prostate cancer. N Engl J Med 2011;364:1995-2005. [Crossref] [PubMed]
- Boudadi K, Antonarakis ES. Resistance to Novel Antiandrogen Therapies in Metastatic Castration-Resistant Prostate Cancer. Clin Med Insights Oncol 2016;10:1-9. [PubMed]
- Terry S, Beltran H. The many faces of neuroendocrine differentiation in prostate cancer progression. Front Oncol 2014;4:60. [Crossref] [PubMed]
- Huang J, Yao JL, di Sant'Agnese PA, et al. Immunohistochemical characterization of neuroendocrine cells in prostate cancer. Prostate 2006;66:1399-406. [Crossref] [PubMed]
- Madu CO, Lu Y. Novel diagnostic biomarkers for prostate cancer. J Cancer 2010;1:150-77. [Crossref] [PubMed]
- Portela-Gomes GM, Lukinius A, Grimelius L. Synaptic vesicle protein 2, A new neuroendocrine cell marker. Am J Pathol 2000;157:1299-309. [Crossref] [PubMed]
- Deftos LJ. Granin-A, parathyroid hormone-related protein, and calcitonin gene products in neuroendocrine prostate cancer. Prostate Suppl 1998;8:23-31. [Crossref] [PubMed]
- Pinto F, Pértega-Gomes N, Vizcaíno JR, et al. Brachyury as a potential modulator of androgen receptor activity and a key player in therapy resistance in prostate cancer. Oncotarget 2016;7:28891-902. [PubMed]
- Wang HT, Yao YH, Li BG, et al. Neuroendocrine Prostate Cancer (NEPC) progressing from conventional prostatic adenocarcinoma: factors associated with time to development of NEPC and survival from NEPC diagnosis-a systematic review and pooled analysis. J Clin Oncol 2014;32:3383-90. [Crossref] [PubMed]
- Goldstein AS, Huang J, Guo C, et al. Identification of a cell of origin for human prostate cancer. Science 2010;329:568-71. [Crossref] [PubMed]
- Stoyanova T, Cooper AR, Drake JM, et al. Prostate cancer originating in basal cells progresses to adenocarcinoma propagated by luminal-like cells. Proc Natl Acad Sci U S A 2013;110:20111-6. [Crossref] [PubMed]
- Beltran H, Rickman DS, Park K, et al. Molecular characterization of neuroendocrine prostate cancer and identification of new drug targets. Cancer Discov 2011;1:487-95. [Crossref] [PubMed]
- Lee JK, Phillips JW, Smith BA, et al. N-Myc Drives Neuroendocrine Prostate Cancer Initiated from Human Prostate Epithelial Cells. Cancer Cell 2016;29:536-47. [Crossref] [PubMed]
- Watson PA, Arora VK, Sawyers CL. Emerging mechanisms of resistance to androgen receptor inhibitors in prostate cancer. Nat Rev Cancer 2015;15:701-11. [Crossref] [PubMed]
- Mosquera JM, Beltran H, Park K, et al. Concurrent AURKA and MYCN gene amplifications are harbingers of lethal treatment-related neuroendocrine prostate cancer. Neoplasia 2013;15:1-10. [Crossref] [PubMed]
- Lotan TL, Gupta NS, Wang W, et al. ERG gene rearrangements are common in prostatic small cell carcinomas. Mod Pathol 2011;24:820-8. [Crossref] [PubMed]
- Pinto F, Pértega-Gomes N, Pereira MS, et al. T-box transcription factor brachyury is associated with prostate cancer progression and aggressiveness. Clin Cancer Res 2014;20:4949-61. [Crossref] [PubMed]