Regulation of cytotoxic T-cell responses by p53 in cancer
Introduction
Tumor suppressor p53 is a transcription factor that has a well characterized role in regulating the expression of numerous downstream target genes involved in cell cycle progression, cell death, and metabolism (1,2). In normal cells, p53’s function is suppressed by its negative regulator murine double minute 2 (MDM2) which induces ubiquitination and degradation of p53 (3). In response to various genotoxic stresses, p53 is post-translationally modified (e.g., phosphorylated), which inhibits the interaction of p53 with MDM2, leading to stabilization and activation of p53 (4).
Increasing evidence indicates that p53 also plays crucial roles in the regulation of immune responses, specifically in several aspects of the cytotoxic T-lymphocyte (CTL)-tumor cell interaction (5-8). Indeed, p53 responsive elements have been found in genes which impact the CTL response including the transporter associated with antigen processing 1 (TAP1) and endoplasmic reticulum amino peptidase 1 (ERAP1), through which p53 is directly involved in the upregulation of antigen presentation via the major histocompatibility complex (MHC) I pathway (5,7) (Figure 1). The interaction between MHC I on cancer cells and the T-cell receptor (TCR) on CTLs is essential for the adaptive immune response to cancer; however, proper co-stimulation is also necessary for the CTL-mediated tumor cell killing (9). The positive co-stimulatory signals required for CTL activation can be trumped by negative regulators [e.g., programmed death-ligand 1 (PD-L1)], if bound to the appropriate receptor (9) (Figure 1). Interestingly, p53 upregulates a microRNA, miR-34, which leads to reduced expression of PD-L1 (6,10) (Figure 1). Also, p53 is known to upregulate Fas/APO-1 expression in tumor cells which results in the induction of CTL-mediated apoptosis upon Fas-ligand (FasL) binding to Fas/APO-1 (8) (Figure 1). Thus, p53 can suppress tumor growth by recruiting CD8+ T-lymphocytes and facilitating their cell killing effects on tumor cells. This review will focus on the interaction of CD8+ T-cells and tumor cells with emphasis on how p53 impacts the outcome of this interaction.
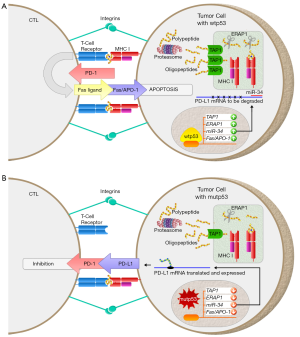
Status of p53 impacts CTL-mediated tumor suppression
CTLs must be activated before inducing apoptosis in a tumor cells. Mature but naive CTLs circulate through the blood stream and lymphatics scanning secondary lymphoid tissues for binding affinity to antigen-MHC I complexes on professional antigen presenting cells (APCs). If the proper co-stimulatory signals are present, the CTL-APC interaction leads to activation of the CD8+ T-cell, which is termed as phase 1 of the CTL response (9). Following phase 1, the CTLs migrate to the site of the tumor. If a tumor cell expresses the antigen-MHC I complex which the CTLs recognize, then phase 2 of the response is initiated.
Phase 2 of the CTL response is an intimate interaction between TCRs on a CTL and antigen-MHC I molecules on a cancer cell. This complex is known as an immunological synapse which is supported by an outer ring of integrin family proteins (9) (Figure 1). Within this synapse, the CTL has two mechanisms which may be utilized to induce apoptosis of the target cell. One is the release of granules which contain perforin and granzymes, and the other is the expression of FasL within this synapse (9). Since both of these mechanisms result in apoptosis without inducing direct lysis of the target cell, it has been said that CTLs don’t kill target cells, they convince them to commit suicide. Expanding on this train of thought, it is now known that tumor cells with wild-type p53 (wtp53) can easily have apoptosis induced by CTLs, whereas tumor cells carrying mutant p53 (mutp53) or lacking wtp53 are resistant to CTL-mediated apoptosis (11).
p53 upregulates factors involved in the MHC I antigen presentation pathway
MHC I is constitutively expressed on nearly all nucleated human cells (9). This integral membrane protein complex is a heterodimer which consists of an α-subunit containing three extracellular domains (α1–α3) and an associated extracellular β2 microglobulin subunit. The α1 and α2 domains form a peptide binding groove which anchors the generally hydrophobic C-terminus of oligopeptides that are 8–10 amino acids in length (12). These oligopeptides are generated by proteasome degradation, expressed extracellularly by MHC I, and evaluated by CTLs through the TCR-MHC I interaction. Therefore, increased expression of MHC I on tumor cells represents an attempt of a cell to flag-down or recruit CTLs in order to evaluate peptides and determine if they are from mutated or foreign proteins. The interferon (IFN) and tumor necrosis factor (TNF) cytokine families are well-documented upregulators of MHC I expression, but intracellular p53 also plays an important role in upregulating the MHC I pathway when there is genotoxic or metabolic stress within the cell, as described in the next section.
p53 upregulates antigen transport in preparation for MHC I loading
Oligopeptides generated via proteasome degradation are initially cytosolic and must be transported into the rough endoplasmic reticulum (ER), in order to be loaded on to MHC I for subsequent expression at the cell surface. TAP1 and TAP2 are the primary proteins that facilitate this transport (13). They are constitutively expressed at low levels and can be upregulated by IFN. Intriguingly, TAP1 expression is also directly upregulated by p53 (7). A p53-responsive element is located approximately 300 nucleotides downstream of the transcription start site of TAP1. Exogenous expression of wtp53 in a p53-null H1299 [human non-small cell lung cancer (NSCLC)] cell line increases TAP1 mRNA expression 4–6 times. This increase in mRNA expression was confirmed by western blotting to result in increased TAP1 protein levels. It was also shown that the common p53 mutants V134A, R175H, R249S, and R273H were unable to bind to this p53 responsive element and consequently unable to increase TAP1 expression (7). This was one of the first clues suggesting that mutations in p53 may contribute to immune evasion by tumor cells.
An important question which Zhu et al. (7) addressed was whether or not upregulation of TAP1 by p53 led to an increase in MHC I-peptide expression at the cell surface. Unfortunately, activation of p53 alone was not sufficient to significantly increase MHC I surface expression in H1299 cells expressing an inducible wtp53. However, when treated with IFNγ for 48 hours, cells expressing wtp53 had a 2.6-fold increase in MHC I expression, as compared with 1.5-fold increase in MHC I in parental p53-null H1299 cells (7). Basal levels of LMP7, a proteasome subunit associated with antigen presentation that responds to IFNγ, are very low in H1299 cells (14), which could explain why the significant increase in MHC I expression is detected only when the H1299 cells are treated with IFNγ (7). Thus, p53 and IFNγ were found to cooperatively upregulate the MHC I antigen presentation pathway in this cell line. However, the later work by Wang et al. (5) shows that p53 activation itself is sufficient to upregulate MHC I without IFNγ treatment in other wtp53-expressing cancer cell lines and hence effector CD8+ T-cells can efficiently respond to mutated or foreign peptides expressed in these cells.
p53 enhances peptide trimming via ERAP1
Over 85% of oligopeptides generated by proteasome degradation are not the proper length to be presented by MHC I (15). As a result, after TAP brings peptides to the ER, ERAP1 needs to further trim these epitopes to fit the MHC I binding cleft (16). This is an essential process in the MHC I antigen presentation pathway, as ERAP1-deficient mice have severely impaired CTL responses due to defective MHC I peptide presentation (17).
Wang et al. (5) demonstrate that p53 is directly involved in the upregulation of ERAP1, further expanding the crucial role of p53 in antigen presentation. By performing a microarray analysis in HCT116 (human colon carcinoma) cells expressing wtp53 and sub-cell lines expressing different p53 mutants (R175H, C176F, G245D, R273C, R280T, R282W), they found that the mRNA expression of TAP1, as well as ERAP1, was higher in the parental HCT116 compared to all of the sub-cell lines expressing mutp53. Quantitative RT-PCR for these genes using p53+/+ and p53−/− HCT116 cells with or without treatment with a p53 activator nutlin-3a also revealed that both TAP1 and ERAP1 mRNAs were upregulated by p53 (5). Further analyses with western blotting and chromatin immunoprecipitation confirmed increase in protein levels of ERAP1 by p53 due to p53 binding to a responsive element in an intron of the ERAP1 gene (5). The Wang’s group (5) classified a more significant role of p53 in MHC I antigen presentation, because the previous work by Zhu et al. (7) observed MHC I upregulation only when p53 activation was combined with IFNγ treatment. However, Wang et al. (5) successfully demonstrated that p53 activation alone led to an increase in MHC I-peptide expression at the cell surface of HCT116 and U2OS cells by flow cytometry.
p53 decreases PD-L1 expression by upregulating miR-34
Inhibition of the interaction between the programmed cell death-1 (PD-1) receptor and one of its ligands, PD-L1, has been demonstrated to be an effective therapy for multiple types of cancer (18). PD-L1 expression in non-lymphoid tissues is associated with T-cell tolerance and the resolution of the immune response (9). This immune checkpoint is beneficial for preventing self-reactive T-cells which would otherwise cause autoimmune disease and is also critical to decrease the inflammatory response after infectious agents have been cleared. However, in the context of malignant neoplasia, PD-L1 expression in tumors inhibits the CTL-mediated killing of tumor cells. PD-L1 overexpression is a common mechanism of immune evasion in cancer and is associated with a poor prognosis of cancer patients (19,20). Intriguingly, wtp53 decreases PD-L1 expression at the tumor cell surface (6,10). This is due to p53’s ability to bind to the promoter region of miR-34 and increase its expression. miR-34 then effectively downregulates PD-L1 through binding to the 3' UTR of PD-L1 mRNA (10,21,22). This p53/miR-34/PD-L1 axis was explored comparing wtp53 with mutp53 in HCT116, H1299, and a primary lung carcinoma (H460) cell lines, as well as in cancer tissues from 181 NSCLC patients (6). All three in vitro systems, including the p53+/+ and p53−/− HCT116 cells treated with nutlin-3, a p53-inducible H1299 cell line, and H460 lung cancer cells with or without knockdown of wtp53, showed that PD-L1 expression was downregulated by p53 via upregulation of miR-34 (6). The authenticity of this pathway was also confirmed through the analysis of cancer tissues from 181 NSCLC patients through data from The Cancer Genome Atlas (TCGA), where a significant inverse correlation between levels of wtp53 and PD-L1 was observed (6). Moreover, tumors with mutp53 had higher levels of PD-L1 than those with wtp53 (6). It should be noted that this TCGA analysis was limited because only mRNA levels, and not protein levels, of p53 and PD-L1 were available (6). Nonetheless, these results indicate that wtp53 binds to the promoter region and increases the mRNA expression of miR-34 which targets the 3' UTR of PD-L1 mRNA; the decreased expression of this immune checkpoint increases the likelihood of CTL-mediated killing of tumor cells (6,9,10,21,22).
p53 in tumors promotes CTL-mediated apoptosis via Fas/APO-1
FasL expression in the immunological synapse during the CTL-tumor cell interaction is a mechanism utilized by CTLs to induce apoptosis of target cells (23). This pathway is dependent upon the expression of the Fas/APO-1 receptor in the cancer cell.
Owen-Schaub et al. (8) first described the upregulation of Fas/APO-1 in tumor cells by p53. Following overexpression of wtp53 in H358 p53-null NSCLC cell line, there was a 2.9- to 3.7-fold increase in Fas/APO-1 protein levels through flow cytometric analyses. This observation was furthermore confirmed by the discovery of p53-responsive elements in the promoter and an intron of the Fas/APO-1 gene (8). Overexpression of several hotspot p53 mutants in p53-null human erythroleukemia cell line K562 failed to upregulate Fas/APO-1 levels. On the other hand, in K562 cells expressing a temperature sensitive mutp53 (V143A), there was an increase in Fas/APO-1 expression at a permissive temperature of 32.5 degrees Celsius (8). Thus, p53 upregulating Fas/APO-1 in cancer cells allows for CTL-mediated apoptosis when the CTL recognizes a foreign or mutated peptide expressed on MHC I.
Discussion
The immunomodulatory functions of p53 have shed new light on why cancer cells are selected for p53 mutations. The loss of p53 function leads to well-described unchecked cell-division and malignant transformation (24). However, these are not the only factors that contribute to the poor prognosis associated with cancers lacking p53 function. As described above, wtp53 upregulates the MHC I antigen presentation pathway, downregulates the inhibitory checkpoint PD-L1 via miR-34, and upregulates the apoptosis receptor Fas/APO-1. Thus, this review article focuses on a less appreciated aspect of this tumor suppressor where loss or mutation of p53 directly leads to an impaired CTL response.
The p53 status of a tumor likely has an influence on the response to therapies which rely upon the CTL response. IL-2 is FDA-approved for the treatment of metastatic melanoma and renal cell carcinoma and is used off-label for neuroblastoma (25); however, no study has examined the response to IL-2 with respect to the p53 mutation status of the tumor. The primary mechanism of action of IL-2 is through the proliferation, differentiation, and recruitment of T-cells (25). However, if a tumor contains mutp53, the lower levels of MHC I and Fas/APO-1, along with increased PD-L1, would likely antagonize the effects of IL-2, hence leading to decreased response rates and uncontested tumor growth. This hypothesis is supported by the observation that the gene transfer of IL-2, along with wtp53, into tumors in a murine mammary adenocarcinoma model shows a synergistic effect on tumor regression (26).
The ability of wtp53 within tumors to enhance the CD8+ T-lymphocyte response defines p53 as an immunostimulatory transcription factor. Given the intricate regulation of the CTL response by p53, it is plausible that p53 regulates other aspects of the adaptive or innate immune responses to cancer which have not been explored. Moreover, it is also possible that mutp53 is actively involved in immunosuppression as a gain-of-function activity. Further investigation is needed to clarify these unexplored hypotheses. In summary, p53 guards the genome in many different ways (27), one of which is by commanding CTLs.
Acknowledgments
The authors thank Mr. James Braun for assistance with graphic design.
Funding: This work was supported by NIH 5R01CA174735-03 (T Iwakuma) grant.
Footnote
Provenance and Peer Review: This article was commissioned by the Guest Editor (Zhi-Min Yuan) for the series “p53 Biology and Cancer” published in Translational Cancer Research. The article has undergone external peer review.
Conflicts of Interest: Both authors have completed the ICMJE uniform disclosure form (available at http://dx.doi.org/10.21037/tcr.2016.11.76). The series “p53 Biology and Cancer” was commissioned by the editorial office without any funding or sponsorship. The authors have no other conflicts of interest to declare.
Ethical Statement: The authors are accountable for all aspects of the work in ensuring that questions related to the accuracy or integrity of any part of the work are appropriately investigated and resolved.
Open Access Statement: This is an Open Access article distributed in accordance with the Creative Commons Attribution-NonCommercial-NoDerivs 4.0 International License (CC BY-NC-ND 4.0), which permits the non-commercial replication and distribution of the article with the strict proviso that no changes or edits are made and the original work is properly cited (including links to both the formal publication through the relevant DOI and the license). See: https://creativecommons.org/licenses/by-nc-nd/4.0/.
References
- Canman CE, Chen CY, Lee MH, et al. DNA damage responses: p53 induction, cell cycle perturbations, and apoptosis. Cold Spring Harb Symp Quant Biol 1994;59:277-86. [Crossref] [PubMed]
- Bensaad K, Vousden KH. p53: new roles in metabolism. Trends Cell Biol 2007;17:286-91. [Crossref] [PubMed]
- Iwakuma T, Lozano G. MDM2, an introduction. Mol Cancer Res 2003;1:993-1000. [PubMed]
- Shieh SY, Ikeda M, Taya Y, et al. DNA damage-induced phosphorylation of p53 alleviates inhibition by MDM2. Cell 1997;91:325-34. [Crossref] [PubMed]
- Wang B, Niu D, Lai L, et al. p53 increases MHC class I expression by upregulating the endoplasmic reticulum aminopeptidase ERAP1. Nat Commun 2013;4:2359. [Crossref] [PubMed]
- Cortez MA, Ivan C, Valdecanas D, et al. PDL1 Regulation by p53 via miR-34. J Natl Cancer Inst 2015;108:djv303 [Crossref] [PubMed]
- Zhu K, Wang J, Zhu J, et al. p53 induces TAP1 and enhances the transport of MHC class I peptides. Oncogene 1999;18:7740-7. [Crossref] [PubMed]
- Owen-Schaub LB, Zhang W, Cusack JC, et al. Wild-type human p53 and a temperature-sensitive mutant induce Fas/APO-1 expression. Mol Cell Biol 1995;15:3032-40. [Crossref] [PubMed]
- Owen JA, Punt J, Stranford SA, et al. Kuby immunology. 7th edition. New York: W. H. Freeman, 2013:109.
- Wang X, Li J, Dong K, et al. Tumor suppressor miR-34a targets PD-L1 and functions as a potential immunotherapeutic target in acute myeloid leukemia. Cell Signal 2015;27:443-52. [Crossref] [PubMed]
- Thiery J, Abouzahr S, Dorothee G, et al. p53 potentiation of tumor cell susceptibility to CTL involves Fas and mitochondrial pathways. J Immunol 2005;174:871-8. [Crossref] [PubMed]
- Ferrington DA, Gregerson DS. Immunoproteasomes: structure, function, and antigen presentation. Prog Mol Biol Transl Sci 2012;109:75-112. [Crossref] [PubMed]
- Leone P, Shin EC, Perosa F, et al. MHC class I antigen processing and presenting machinery: organization, function, and defects in tumor cells. J Natl Cancer Inst 2013;105:1172-87. [Crossref] [PubMed]
- Nandi D, Jiang H, Monaco JJ. Identification of MECL-1 (LMP-10) as the third IFN-gamma-inducible proteasome subunit. J Immunol 1996;156:2361-4. [PubMed]
- Kisselev AF, Akopian TN, Woo KM, et al. The sizes of peptides generated from protein by mammalian 26 and 20 S proteasomes. Implications for understanding the degradative mechanism and antigen presentation. J Biol Chem 1999;274:3363-71. [Crossref] [PubMed]
- Alvarez-Navarro C, López de Castro JA. ERAP1 structure, function and pathogenetic role in ankylosing spondylitis and other MHC-associated diseases. Mol Immunol 2014;57:12-21. [Crossref] [PubMed]
- Yan J, Parekh VV, Mendez-Fernandez Y, et al. In vivo role of ER-associated peptidase activity in tailoring peptides for presentation by MHC class Ia and class Ib molecules. J Exp Med 2006;203:647-59. [Crossref] [PubMed]
- Shien K, Papadimitrakopoulou VA, Wistuba II. Predictive biomarkers of response to PD-1/PD-L1 immune checkpoint inhibitors in non-small cell lung cancer. Lung Cancer 2016;99:79-87. [Crossref] [PubMed]
- Nakanishi J, Wada Y, Matsumoto K, et al. Overexpression of B7-H1 (PD-L1) significantly associates with tumor grade and postoperative prognosis in human urothelial cancers. Cancer Immunol Immunother 2007;56:1173-82. [Crossref] [PubMed]
- Gao Q, Wang XY, Qiu SJ, et al. Overexpression of PD-L1 significantly associates with tumor aggressiveness and postoperative recurrence in human hepatocellular carcinoma. Clin Cancer Res 2009;15:971-9. [Crossref] [PubMed]
- Chang TC, Wentzel EA, Kent OA, et al. Transactivation of miR-34a by p53 broadly influences gene expression and promotes apoptosis. Mol Cell 2007;26:745-52. [Crossref] [PubMed]
- Raver-Shapira N, Marciano E, Meiri E, et al. Transcriptional activation of miR-34a contributes to p53-mediated apoptosis. Mol Cell 2007;26:731-43. [Crossref] [PubMed]
- Lettau M, Paulsen M, Schmidt H, et al. Insights into the molecular regulation of FasL (CD178) biology. Eur J Cell Biol 2011;90:456-66. [Crossref] [PubMed]
- Iwakuma T, Lozano G. Crippling p53 activities via knock-in mutations in mouse models. Oncogene 2007;26:2177-84. [Crossref] [PubMed]
- Aldesleukin. Drug Information. 2016. Available online: www.lexi.com
- Pützer BM, Bramson JL, Addison CL, et al. Combination therapy with interleukin-2 and wild-type p53 expressed by adenoviral vectors potentiates tumor regression in a murine model of breast cancer. Hum Gene Ther 1998;9:707-18. [Crossref] [PubMed]
- Cheok CF, Verma CS, Baselga J, et al. Translating p53 into the clinic. Nat Rev Clin Oncol 2011;8:25-37. [Crossref] [PubMed]