Potential of Akt mediated DNA repair in radioresistance of solid tumors overexpressing erbB-PI3K-Akt pathway
Introduction
Radiotherapy is one of the major modalities of cancer treatment used to treat approximately 50% of all cancer patients with varying success. The dose of irradiation that can be given to the tumor is determined by the radiosensitivity of surrounding normal tissues (1) as well as intrinsic sensitivity/resistance of tumors. Resistance to radiotherapy can be either intrinsic radioresistance or an acquired resistance during fractionated radiotherapy. One of the molecular events by which tumors can become radioresistant is radiation-induced and potentially ligand-independent activation of signal transduction pathways such as those regulated by membrane-bound receptor tyrosine kinases (RTKs). In this context the role of epidermal growth factor receptor (EGFR) has been extensively investigated. In tumor cells activation of EGFR stimulates signal transduction pathways that ultimately promote tumor cell proliferation, survival, invasion, and angiogenesis (2,3). This leads to both chemo- and radiotherapy resistance and consequently to a poor prognosis (4-6). The pro-survival effect of EGFR is mediated by activation of various downstream signaling pathways, such as the phosphatidyl-inositol 3-kinase (PI3K)/Akt pathway, the signal transducer and activator of transcription (STAT) pathway and the Ras-mitogen-activated protein kinase (MAPK) pathway (7-9). Among the pathways activated by RTKs, PI3K/Akt pathway is the major survival pathway in cancer cells, which is frequently upregulated in human tumors (10,11). PI3K/Akt pathway is constitutively active in tumor cells presenting mutation in one of the components of the EGFR downstream pathways, such as phosphatase and tensin homolog (PTEN), PIK3CA and RAS (12,13). Mutational activation of RAS and PI3K is accompanied with resistance to radio-/chemotherapy (14-17). Thus, the activation status of the PI3K pathway as a consequence of overexpression of RTKs or mutations of the signaling components might be a predictive marker for the response of tumor cells to radiotherapy. Akt, which is also known as protein kinase B (PKB), is a central transducer protein downstream of PI3K and is involved in a number of important cellular processes including cell growth, proliferation, survival, invasion, metastasis, and angiogenesis (18). In addition to these well-described functions, accumulating evidence indicates that Akt is directly involved in the control of DNA repair and radioresistance. In this review, the expression and activity status of Akt isoforms in cancers from different origins will be summarized. We also review the role of Akt family members, especially, Akt1 in radioresistance of solid tumors, and briefly summarize the role of activated Akt in the context of DNA repair.
Role of Akt/PKB in human cancers
Akt/PKB is a serine/threonine kinase, which consists of three isoforms known as Akt1 (PKBα), Akt2 (PKBβ) and Akt3 (PKBγ). These isoforms are products of different genes with more than 80% homology on chromosomes 14q32, 19q13 and 1q44, respectively (19). Different isoforms of Akt have different functions in normal physiology and development (20). Akt1 is required for normal growth (21) and mammary morphogenesis/function (22). Akt2 is essential for glucose metabolism, adipogenesis and β-cell function (23,24) and, as so far known, Akt3 is essential for attainment of normal brain size (25). For Akt over 40 downstream targets have been reported, which contribute to the cellular roles of Akt, including cell survival, growth, proliferation, angiogenesis, metabolism, and migration (26).
In human malignancies, Akt activity plays a major role in tumor cell survival (27). Enhanced activation of Akt is one of the common molecular disregulations due to AKT gene amplification, amplification-independent overexpression and hyperactivation of Akt through activation of upstream pathways (28). Hyperactivation of Akt is a common mechanism of increased cell survival, proliferation and aggressiveness in tumors presenting overexpression of Akt upstream components such as expression of a specific EGFR mutant (EGFRvIII, also known as EGFR type III, de2-7, Delta EGFR) (29-31). Mutation in RAS family members, i.e. in K-RAS and H-RAS, also stimulate Akt phosphorylation and tumor cell survival (32-36) mainly through upregulated production of EGFR-ligands such as amphiregulin and transforming growth factor α. Additionally, in tumors from different origins point mutations, loss of heterozygosity or methylation of PTEN (a phosphatase that deactivates PI3K) as one of the most frequently inactivated tumor suppressor genes (37) leads to activation of downstream components of PI3K signaling (38,39) including Akt. Immunostaining of ovarian cancer samples revealed an inverse correlation between PTEN expression and the phosphorylation level of Akt (40). Likewise, loss of PTEN is associated with worse clinical outcome as shown for patients with esophageal adenocarcinomas (41).
Previous reports indicate that amplification, mutation, or overexpression of Akt in tumors from different origins are Akt isoform-specific phenomena (28) which result in Akt hyperactivation. Hyperactivation of Akt correlates with various clinicopathologic parameters and is a prognostic indicator for cancers from different origins such as oral, pancreatic, thyroid and lung cancers (42-45). Amplification of AKT1 in general is a rare phenomenon in cancers, which was demonstrated for the first time in a primary human gastric adenocarcinoma (46) and later in a small population of estrogen receptor-positive breast carcinomas and prostate cancers (47). A somatic AKT1-E17K mutation has been reported in different cancers such as in breast; high-grade endometrial, bladder, lung and colorectal cancers (48-51). Similar to hotspot mutation, several non-hotspot mutations in AKT1 have also been identified, which cause a constitutive membrane localization of Akt1. This results in a permanent Akt1 kinase activity and phosphorylation at T308 and S473 and consequent activation of downstream target proteins independent of growth factor stimulation (52). Mutation in AKT1 (Glu17Lys) can also regulate tumorigenesis (51).
Similar to Akt1, Akt2 through amplification and overexpression exerts oncogenic activity as well. For the full activation, Akt2 needs to be phosphorylated on T309 and S474 (53). Amplification of Akt2 is more frequent than amplification of Akt1 and has been demonstrated for human malignancies such as pancreatic, colorectal, ovarian and breast cancers (54-60). Interestingly, in ovarian cancer cells Akt1 is phosphorylated/activated and the activation/phosphorylation is Ras-, src- and PI3K-dependent when cells are stimulated with growth factors (61). Mutation analyses of AKT2 revealed that somatic mutations in AKT2 kinase domain can occur in a subset of stomach, lung, colon and breast cancers. These observations indicate that alterations in various signaling cascades depending on mutated AKT2 contribute to tumorigenesis (62).
AKT3 amplification and overexpression may play a role in the genesis of different cancers as described for a subset of ovarian cancers through modulation of cell cycle progression (63), thyroid cancer (45) and the estrogen receptor-negative breast cancers as well as androgen- insensitive prostate carcinomas (64). But so far, the most studies for the carcinogenic role of Akt3 and its impact on treatment outcome have been performed in melanoma (65,66). For the full activity, Akt3 needs to be phosphorylated at S472 and T305 (67). Similar to AKT1 (see above) an E17K mutation in AKT3 gene leads to a constitutive activity of Akt3 (66) and stimulation of substrates such as PRAS40. As reported by Madhunapantula and colleagues (68), this leads to an Akt3-dependent survival advantage and resistance to chemotherapy of melanomas (68).
Importance of Akt in radioresistance
A significant correlation between phosphorylated EGFR and phosphorylated Akt (69) indicates that EGFR is the major regulator of activation of PI3K/Akt pathway. Overexpression or mutation of EGFR has been reported in 40% to 50% of human solid tumors (70,71). Preclinical and clinical studies demonstrated that the hyperactivation of EGFR leads to both chemo- and radiotherapy resistance and consequently to a poor prognosis (4,6,31,63,72-75). Similar to ligand stimulations, exposure to ionizing radiation induces activation of EGFR and its downstream PI3K/Akt pathway (76-80). PI3K/Akt activity regulates cell growth, proliferation and survival, which will influence the response to ionizing radiation. The function of PI3K/Akt activity on radioresistance has been reported by several laboratories in different cancer cell lines including those from head and neck, colon, lung, brain cancers in vitro (36,76,77,80-82) independent of TP53 status (83) as well as in vivo (84). Although, stimulation of PI3K, in parallel to increased Akt activity, regulates the activation of other pro-survival substrates such as SGK (10), it has been demonstrated that Akt activation downstream of PI3K plays a major role in the radiation resistance of tumor cells with different entities. In this context Brognard and colleagues (85) reported that a constitutive phosphorylation of Akt, presumably Akt1, at S473 and T308 mediates radiotherapy resistance in NSCLC cells (85). In this study (85) and studies from others (34,80,84,86-89) radiosensitization was shown to be achieved either by pharmacological targeting of PI3K/Akt or genetic modulation of Akt. In a similar study conducted in bile duct cancer (BDC), Tanno et al. (90) described the expression of phosphorylated Akt at S473 in 84.2% of patients with BDC. Using BDC cell lines in vitro, these authors demonstrated that phosphorylation of Akt depends on PI3K activity (90). Targeting PI3K by LY294002 led to a similar degree of radiosensitization as observed in cells carrying a dominant-negative Akt (90). From these studies it can be concluded that Akt is a major mediator of PI3K-induced cellular resistance to radiotherapy. This conclusion is supported by reduced radiation sensitivity following overexpression of constitutively active Akt into tumor cells from different origins such as NSCLC (85), bile duct cancer (90) and colon cancer (40). In line with these preclinical findings, the prognostic value of activated Akt for the radiation response of solid tumors has been described in clinical investigations as well. Immunohistochemical analysis of phosphorylated Akt1 at S473 implies that activated Akt1 is a potential predictive biomarker for the radiotherapy response as shown for patients with head and neck cancer (91) as well as patients with locally advanced cervical cancer (92). Although, the pro-survival effect of Akt activity in oncology is well accepted, the specific mechanism by which Akt improves cell survival, especially after radiotherapy, has not been understood so far.
It has been postulated that inhibition of apoptosis is one of the mechanisms by which activated Akt improves cell survival. To inhibit apoptosis, Akt phosphorylates and inactivates pro-apoptotic proteins such as BAD, BAX and caspase-9. Akt-induced BAD phosphorylation prevents binding of BAD to BCL2 family members (93). Likewise, stimulating Akt upregulates the expression level of several intracellular antiapoptotic proteins such as FLIP, survivin, cIAP1; cIAP-2, A1/Bfl-1, and XIAPs (94,95). Akt-mediated inhibition of apoptosis abrogates the sensitivity of hematopoietic cells such as leukemia cells to therapeutic approaches inducing apoptosis (95). Ionizing radiation can also induce apoptosis through mitochondria-dependent intrinsic pathway (96). In this pathway, activated Akt phosphorylates proapoptotic proteins BAX and BAD. Phosphorylation of Bax at S184 which is regulated in Akt-dependent manner inhibits Bax effects on the mitochondria by maintaining the protein in the cytoplasm (97). Yet, heterogeneous effects of Akt inhibition on radiation-induced apoptosis have been reported. We could show that neither in apoptosis-sensitive nor in apoptosis resistant NSCLC cell lines targeting Akt does results in enhanced radiation-induced apoptosis. In both cell types, Akt targeting led to an inhibition of repair of radiation-induced DNA-DSB and subsequent enhancement of radiation sensitivity (98). In this context it has also been demonstrated that inhibition of Akt in malignant gliomas mediates radiosensitization which is independent of apoptosis (99). Although, in a previous report by Tanno et al. (90), inhibition of Akt was associated with increased apoptosis, this study did not investigate impact of apoptosis on radiosensitization (90). Thus, the role of apoptosis as a mechanism of cell death in radiosensitization mediated through Akt targeting in human solid tumors is rather questionable and needs further investigations.
Experimental and clinical evidence indicates that cancer-initiating cells or cancer stem cells (CSC) are resistant to radiotherapy (100). In this context CD44 expression bears the potential to predict outcome of radiotherapy by assessment of CSC density (100). Moreover, it has been demonstrated that the expression of AKT1 and AKT2 after irradiation is increased in the breast cancer MCF-7 mammospheres CD24(-/low)/CD44(+) expressing cells, but not in the bulk population of MCF-7 CD24(+)/CD44(+) expressing cells (101). In this study (101) tar-geting of Akt did sensitize MCF-7 mammosphere cells, but not MCF-7 monolayer cells to ionizing radiation. Thus, it seems that Akt through a so far unknown mechanism is involved in radioresistance of CSC. The role of Akt activity in radioresistance of CSC has been demonstrated in both mammospheres in vitro as well as in syngeneic mice bearing tumors in vivo (102). Zhang et al. in this study (102) reported that inhibition of the Akt pathway inhibits ca-nonical Wnt signaling as well as repair of DNA damage selectively in cancer initiating cells and sensitizes them to ionizing radiation in vitro and in vivo (102). With respect to the described functional role of PI3K/Akt pathway in CSC (99,103), inhibition of the Akt pathway might offer an improved radiation response of CSC (101,102). Based on the assumption that CSC are more radioresistant and present hyperactivation of the Akt pathway, we were able to show that selected radioresistant subpopulations of A549 cells which present the CSC marker ALDH1 can be radiosensitized by PI3K inhibitor LY294002 (104). The importance of PI3K/Akt signaling for radioresistance of CSC is also underlined by the data of Zhang et al. (102) indicating Akt dependence of accelerated repair of radiation-induced DNA-DSB
In recent years autophagy has been recognized as an important process in carcinogenesis as well as in processes mediating the response of tumor cells to therapy, especially radiation therapy (105,106). Clear evidence exists that PI3K/Akt signaling plays an important role in the regulation of autophagy. Exposure of tumor cells to ionizing radiation induces autophagy and previous reports indicate that inhibition of autophagy either by autophagy inhibitors (107) or genetic approaches (105) induces radiosensitization. In contrast to the cytoprotective effect of radiation-induced autophagy, induction of autophagy via the PI3K/Akt/mTOR pathway is a cytotoxic effect. This is supported by the radiosensitizing effect of Akt inhibition in malignant glioma cells through inducing autophagy (108). Further investigations are needed to identify the mechanism(s) involved in the cytoprotective effect of radiation-induced autophagy and cytotoxic effect of Akt induced autophagy on post-irradiation survival.
Control of DNA double-strand break repair by Akt as one major mechanism of radioresistance and a possible specific target for radiosensitization in tumor cells
Previous reports indicate a direct correlation between EGFR as the major regulator of Akt activity and DNA-DSB repair (74,109-111). Moreover, impact of Akt activity on DNA-DSB repair and radioresistance in tumor cells from different origins has been demonstrated as well (15,79,98,112,113). DNA-DSBs are the most lethal type of DNA lesions that lead to cell death following exposure to ionizing radiation (114). Two pathways are involved in DNA-DSBs repair, non-homologous end-joining (NHEJ) and homologous recombination (HR) (115), but NHEJ is the predominant pathway in mammals. The DNA-dependent protein kinase catalytic subunit complex (DNA-PKcs) is a major enzyme in the NHEJ process and ra-diotherapy response (116,117). Phosphorylation of DNA-PKcs at specific amino acid residues including T2609 cluster and S2056 are required and essential for efficient repair of DNA-DSBs during NHEJ (118). Enhanced cellular sensitivity to IR after mutations in these phosphorylation sites supports the specific function of DNA-PKcs phosphorylation in DNA-DSBs repair (119-121). Previous reports from our laboratory (98,112) and the report by Park and colleagues (122) demonstrated that Akt directly interacts with DNA-PKcs through its C-terminal domain. Akt1 and DNA-PKcs form a functional complex after radiation exposure (112,123). Using GFP-tagged DNA-PKcs expressing cells, we were able to demonstrate for the first time that Akt1 promotes DNA-PKcs accumulation at the DNA-DSB site and stimulates DNA-PKcs kinase activity which is the necessary step for progression of DNA-DSB repair (112). Akt1 dependent DNA-PKcs kinase activity results in DNA-PKcs autophosphorylation at S2056 (112) which is essential for efficient repair (121) as well as the release of DNA-PKcs from the damage site. The role of Akt in DNA-DSB repair is further substantiated by co-localization γH2AX foci with P-Akt after irradiation (112,113,123,124). Based on these results, a detailed mechanism of activation of DNA-PKcs by Akt (summarized in Figure 1) can be proposed. Akt activity through mutation or overexpression of upstream components such as erbB receptors (30,79,109,125), PTEN (15,126), RAS (35,88,127) and PI3K (109) results in increased radiation-induced DNA-PKcs activity and enhance repair of DNA-DSB. Thus, in the case of dysregulation of these molecules such as mutated PI3K direct targeting of PI3K or inhibition of downstream components like Akt, but not targeting of upstream components such as EGFR, can result in radiosensitization. Based on this argument, it is proposed that targeting of PI3K in combination with radiotherapy leads to heterogeneous responses depending on the Akt genotype, i.e., radiosensitization in Akt wild-type tumor cells and the lack of radiosensitization in Akt-mutated tumor cells.
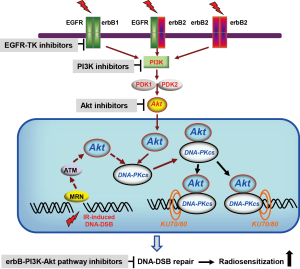
An alternative pathway regulating DNA-DSB repair Akt is the upregulation of MRE11 expression after Akt activation through Akt/GSK3β/β-catenin/LEF-1 pathway (117). MRE11 is a central protein which binds to RAD50 and NBS1. It has been proven that the MRE11, RAD50 and NBS1 (MRN) complex after irradiation rapidly accumulates to damage site and remains until the damage has been repaired. MRN complex appears to be the major sensor of the breaks and subsequently recruits ATM where it is activated to phosphorylate in turn members of that complex and a variety of other proteins involved in cell-cycle control and DNA repair (128). Approximately 85% of DNA-DSBs induced by ionizing radiation are repaired within the first 2-3 h post-irradiation via the fast component of DNA-DSB repair, which has been shown to be independent of ATM function (129). The remaining 15% of DNA-DSBs, which are mainly composed of complex lesions, are repaired in an ATM-dependent manner via the slow component (130,131). Since targeting Akt leads to downregulation of MRE11 at the transcriptional level, role of Akt1 on DNA repair under this mechanism might be due to regulating slow components of DNA repair and is a complementary mechanism to Akt/DNA-PKcs dependent fast repair process. As reported by Viniegra et al. (132), full activation of Akt in response to insulin and ionizing radiation is ATM-dependent. In this context Fraser and colleagues (113) showed that the activation of MRE11-ATM-RNF168 pathway induce Akt phosphorylation and this leads to an Akt-dependent enhanced repair of DNA-DSB repair (113). In line with the role of ATM in Akt activity after irradiation (133), it has been reported that ATM-dependent Akt signaling regulates DNA-DSB repair in cells exposed to clinical relevant doses of irradiation (134,135). The dependence of DNA-PKcs activity through transphosphorylation at T2609 on ATM (121) as well as Akt (88,98) indicates that activation of Akt/DNA-PKcs in the nucleus can at least partially be ATM-dependent (Figure 1). Together, Akt-dependent activation/phosphorylation of DNA-PKcs (88,98,112) indicates that Akt is involved in the fast component of DNA-DSBs repair. Likewise, since ATM phosphorylates Akt (133) and Akt activity upregulates MRE11 (117) which is a rather slow process, it can be concluded that Akt is also part of the slow component of DNA-DSB repair. These two aspects needs to be further investigated.
In contrast to NHEJ, which in general is active throughout the cell cycle and does not rely on a template, HR is restricted to the S- and G2-phases of the cell cycle only due to the requirement for a sister chromatid as template: while the balance between both pathways is essential for genome stability, disturbance of that balance can lead to cancer (136). BRCA1, BRCA2 and RAD51 are the major proteins regulating HR repair. As described for hereditary breast and ovarian cancers, BRCA1 and BRCA2 are frequently mutated in these cancers (137,138) which might be due to genomic instability as a consequence of HR deficiency. A link between Akt1 activity and HR has also been demonstrated. HR deficiency is associated with increased phosphorylation of Akt1 at S743 as shown in breast cancer patients. Likewise, tumor formation by BRCA1 deficiency is reduced by Akt1 depletion (139). Inhibition of HR by Akt1 activity in BRCA1 proficient breast cancer cells has been demonstrated to be due to induction of cytoplasmic retention of BRCA1 and RAD51 (140). In HR-deficient cells, Akt1 mediated inhibition of HR is through impaired Chk1 nuclear localization and subsequently disruption of Chk1-Rad51 interaction (141). Thus, from these studies it becomes clear that in contrast to the stimulatory effect of Akt1 on NHEJ, in tumor cells aberrant activation of Akt inhibits HR and generates genetic instability (140). Regarding the role of Akt activity regulated by PTEN in DNA-DSB repair conflicting reports exist. Puc et al. (142) have demonstrated that PTEN knockdown generates DNA damage due to insufficient inactivation of CHK1 un-der non-irradiated basal condition. In this report by Puc et al. (142), although γH2AX foci assay in control-siRNA transfected and irradiated cells was used as positive control, the effect of PTEN-siRNA on residual DNA-DSB was not investigated. Thus, this study does not provide strong evidence that inhibition of HR is mediated through Akt activity. With respect to the role of PTEN in DNA-DSB repair, Kao et al. (15) demonstrated that overexpression of PTEN results in decreased Akt1 phosphorylation and increased residual DNA-DSB. The study and results by Kao et al. do not support the role of Akt activity in HR as suggested by other groups (139-141), i.e. that reduced Akt activity following PTEN overexpression leads to an increase in HR and consequently accelerated repair of DNA-DSB. Overexpression of PTEN, associated with reduced Akt activation, resulted in an impaired repair of DNA-DSB (15). Since the effect of Akt on DNA-DSB repair is a combination of both NHEJ and HR repair pathways, it can be assumed that the effect of Akt stimulated repair of DNA-DSB by NHEJ is dominant over Akt mediated impairment of DNA-DSB repair in HR-deficient cells. A study by Li et al. (143) indicates that EGFR tyrosine kinase inhibitor erlotinib attenuates homologous recombinational repair of chromosomal breaks in human breast cancer cells after irradiation (143). Since erlotinib inhibits Akt phosphorylation, from this study as well as from a study by Kao et al. (15) it may be concluded that Akt has a differential effect on HR after irradiation on tumor cells compared to non-irradiated condition. Nevertheless, the distinct role of Akt in repair of DNA-DSB through the HR pathway needs to be further investigated.
The reports discussed in this chapter underline the role of Akt in repair of DNA-DSB via NHEJ repair process. So far, to our knowledge no clinical study combining Akt inhibitor treatment with radiotherapy has been conducted. However, data obtained for the combination of dual PI3K and mTOR inhibitors such as BEZ235 with radiotherapy in animal systems suggest that inhibition of PI3K/Akt pathway is an effective strategy to improve radiotherapy, especially in tumors with K-RAS mutation (144). This study also indicates the importance of constitutive Akt activity stimulated in K-RAS mutated cells for effective DNA-DSB repair.
With respect to the approach to use the inhibitors of NHEJ pathway to improve radiotherapy of tumors, the effect on normal tissue should not be ignored. Since the NHEJ repair pathway is also the major pathway for repair of DNA-DSB in normal cells; targeting of the components of this pathway will inhibit repair of DNA-DSB simultaneously in tumor cells as well as in normal cells. Therefore, in spite of efficient radiosensitization by using targeting of the components of NHEJ repair pathway such as DNA-PKcs, molecular targeting of NHEJ due to normal tissue damage cannot be applied to improve radiotherapy. Since dysregulations of PI3K/Akt pathway due to mutation, gene amplification or overexpression is a tumor-specific phenomenon; inhibition of Akt-dependent DNA repair pathway might be a selective approach to kill tumor cells.
Akt inhibitors are either ATP mimetics or allostric inhibitors which bind to the pleckstrin-homology domain of Akt. The current clinical trials in oncology are testing the feasibility and applicability of these inhibitors such as Perifosine, MK2206 and Nelfinavir (allosteric Akt inhibitor) or GSK690693 (ATP-competitive inhibitor) (10,145). Most of the so far described Akt inhibitors target all 3 Akt isoforms. Depending on the outcome of clinical trials in oncology with the Akt inhibitors, Akt targeting strategies, especially, targeting of Akt1 prior to radiotherapy might be highly effective to overcome PI3K/Akt-dependent radioresistance of solid tumors.
Conclusions
Molecular targeting approaches in oncology are based on understanding the function of cellular signaling pathways in tumor growth, proliferation and survival. Activation of EGFR/PI3K/Akt signaling pathway is crucial for post-irradiation cell survival. Most of the small-molecule inhibitors used for targeting signal components within this pathway are cytostatic rather than cytotoxic. Likewise, hyperactivation of the downstream components of this pathway such as mutations in PTEN, PI3K, or Akt related genes results in a lack of response to inhibitors of upstream molecules, e.g., EGFR. Although EGFR is the major regulator of the PI3K/Akt pathway, this pathway can also be regulated by many other receptors like G-protein coupled receptors, integrin receptors and insulin receptors. Thus, based on the preclinical studies combining Akt inhibitors with radiotherapy can shift the cytostatic effect of Akt inhibitors towards an increased cytotoxicity of radiotherapy. Likewise, as a consequence of targeting Akt as the key player of PI3K/Akt signaling, the crosstalk between several membrane-bound receptors cannot result in reactivation of Akt signaling.
Thus, based on the described role of Akt in repair of DNA-DSB, Akt rather than EGFR or PI3K should be the prime molecule to be targeted in order to overcome radiotherapy resistance of solid tumors presenting enhanced PI3K/Akt signaling.
Acknowledgments
Funding: This work was supported by grants from the Deutsche Forschungsgemeinschaft [Ro527/5-1 and SFB-773-TP B02] awarded to HPR, GRK 1302/2 (T11) awarded to MT/HPR.
Footnote
Provenance and Peer Review: This article was commissioned by the Guest Editors (David J. Chen and Benjamin P.C. Chen) for the series “DNA Damage and Repair” published in Translational Cancer Research. The article has undergone external peer review.
Conflicts of Interest: Both authors have completed the ICMJE uniform disclosure form (available at http://dx.doi.org/10.3978/j.issn.2218-676X.2013.04.09). The series “DNA Damage and Repair” was commissioned by the editorial office without any funding or sponsorship. The authors have no other conflicts of interest to declare.
Ethical Statement: The authors are accountable for all aspects of the work in ensuring that questions related to the accuracy or integrity of any part of the work are appropriately investigated and resolved.
Open Access Statement: This is an Open Access article distributed in accordance with the Creative Commons Attribution-NonCommercial-NoDerivs 4.0 International License (CC BY-NC-ND 4.0), which permits the non-commercial replication and distribution of the article with the strict proviso that no changes or edits are made and the original work is properly cited (including links to both the formal publication through the relevant DOI and the license). See: https://creativecommons.org/licenses/by-nc-nd/4.0/.
References
- Begg AC, Stewart FA, Vens C. Strategies to improve radiotherapy with targeted drugs. Nat Rev Cancer 2011;11:239-53. [PubMed]
- Nyati MK, Morgan MA, Feng FY, et al. Integration of EGFR inhibitors with radiochemotherapy. Nat Rev Cancer 2006;6:876-85. [PubMed]
- Huang SM, Harari PM. Modulation of radiation response after epidermal growth factor receptor blockade in squamous cell carcinomas: inhibition of damage repair, cell cycle kinetics, and tumor angiogenesis. Clin Cancer Res 2000;6:2166-74. [PubMed]
- Ang KK, Berkey BA, Tu X, et al. Impact of epidermal growth factor receptor expression on survival and pattern of relapse in patients with advanced head and neck carci-noma. Cancer Res 2002;62:7350-6. [PubMed]
- Krause M, Zips D, Thames HD, et al. Preclinical evaluation of molecular-targeted anti-cancer agents for radiotherapy. Radiother Oncol 2006;80:112-22. [PubMed]
- Nakamura JL. The epidermal growth factor receptor in malignant gliomas: pathogenesis and therapeutic implications. Expert Opin Ther Targets 2007;11:463-72. [PubMed]
- Rodemann HP, Dittmann K, Toulany M. Radiation-induced EGFR-signaling and control of DNA-damage repair. Int J Radiat Biol 2007;83:781-91. [PubMed]
- Rodemann HP, Blaese MA. Responses of normal cells to ionizing radiation. Semin Radiat Oncol 2007;17:81-8. [PubMed]
- Toulany M, Rodemann HP. Membrane receptor signaling and control of DNA repair after exposure to ionizing radiation. Nuklearmedizin 2010;49:S26-30. [PubMed]
- Liu P, Cheng H, Roberts TM, et al. Targeting the phosphoinositide 3-kinase pathway in cancer. Nat Rev Drug Discov 2009;8:627-44. [PubMed]
- Liu Z, Roberts TM. Human tumor mutants in the p110alpha subunit of PI3K. Cell Cycle 2006;5:675-7. [PubMed]
- Cengel KA, Voong KR, Chandrasekaran S, et al. Oncogenic K-Ras signals through epidermal growth factor receptor and wild-type H-Ras to promote radiation survival in pancreatic and colorectal carcinoma cells. Neoplasia 2007;9:341-8. [PubMed]
- McKenna WG, Muschel RJ, Gupta AK, et al. The RAS signal transduction pathway and its role in radiation sensitivity. Oncogene 2003;22:5866-75. [PubMed]
- Sekhar KR, Reddy YT, Reddy PN, et al. The novel chemical entity YTR107 inhibits recruitment of nucleophosmin to sites of DNA damage, suppressing repair of DNA double-strand breaks and enhancing radiosensitization. Clin Cancer Res 2011;17:6490-9. [PubMed]
- Kao GD, Jiang Z, Fernandes AM, et al. Inhibition of phosphatidylinositol-3-OH ki-nase/Akt signaling impairs DNA repair in glioblastoma cells following ionizing radia-tion. J Biol Chem 2007;282:21206-12. [PubMed]
- Misale S, Yaeger R, Hobor S, et al. Emergence of KRAS mutations and acquired resistance to anti-EGFR therapy in colorectal cancer. Nature 2012;486:532-6. [PubMed]
- Garrido-Laguna I, Hong DS, Janku F, et al. KRASness and PIK3CAness in patients with advanced colorectal cancer: outcome after treatment with earlyphase trials with targeted pathway inhibitors. PLoS One 2012;7:e38033 [PubMed]
- Cheung M, Testa JR. Diverse mechanisms of AKT pathway activation in human malignancy. Curr Cancer Drug Targets 2013;13:234-44. [PubMed]
- Murthy SS, Tosolini A, Taguchi T, et al. Mapping of AKT3, encoding a member of the Akt/protein kinase B family, to human and rodent chromosomes by fluorescence in situ hybridization. Cytogenet Cell Genet 2000;88:38-40. [PubMed]
- Dummler B, Hemmings BA. Physiological roles of PKB/Akt isoforms in development and disease. Biochem Soc Trans 2007;35:231-5. [PubMed]
- Cho H, Thorvaldsen JL, Chu Q, et al. Akt1/PKBalpha is required for normal growth but dispensable for maintenance of glucose homeostasis in mice. J Biol Chem 2001;276:38349-52. [PubMed]
- LaRocca J, Pietruska J, Hixon M. Akt1 is essential for postnatal mammary gland development, function, and the expression of Btn1a1. PLoS One 2011;6:e24432 [PubMed]
- Fischer-Posovszky P, Tews D, Horenburg S, et al. Differential function of Akt1 and Akt2 in human adipocytes. Mol Cell Endocrinol 2012;358:135-43. [PubMed]
- Cho H, Mu J, Kim JK, et al. Insulin resistance and a diabetes mellitus-like syndrome in mice lacking the protein kinase Akt2 (PKB beta). Science 2001;292:1728-31. [PubMed]
- Easton RM, Cho H, Roovers K, et al. Role for Akt3/protein kinase Bgamma in attainment of normal brain size. Mol Cell Biol 2005;25:1869-78. [PubMed]
- Manning BD, Cantley LC. AKT/PKB signaling: navigating downstream. Cell 2007;129:1261-74. [PubMed]
- Bellacosa A, Kumar CC, Di Cristofano A, et al. Activation of AKT kinases in cancer: implications for therapeutic targeting. Adv Cancer Res 2005;94:29-86. [PubMed]
- Altomare DA, Testa JR. Perturbations of the AKT signaling pathway in human cancer. Oncogene 2005;24:7455-64. [PubMed]
- Hatanpaa KJ, Burma S, Zhao D, et al. Epidermal growth factor receptor in glioma: signal transduction, neuropathology, imaging, and radioresistance. Neoplasia 2010;12:675-84. [PubMed]
- Mukherjee B, McEllin B, Camacho CV, et al. EGFRvIII and DNA double-strand break repair: a molecular mechanism for radioresistance in glioblastoma. Cancer Res 2009;69:4252-9. [PubMed]
- Li B, Yuan M, Kim IA, et al. Mutant epidermal growth factor receptor displays increased signaling through the phosphatidylinositol-3 kinase/AKT pathway and promotes radioresistance in cells of astrocytic origin. Oncogene 2004;23:4594-602. [PubMed]
- Toulany M, Baumann M, Rodemann HP. Stimulated PI3K-AKT signaling mediated through ligand or radiation-induced EGFR depends indirectly, but not directly, on constitutive K-Ras activity. Mol Cancer Res 2007;5:863-72. [PubMed]
- Toulany M, Schickfluss TA, Eicheler W, et al. Impact of oncogenic K-RAS on YB-1 phosphorylation induced by ionizing radiation. Breast Cancer Res 2011;13:R28. [PubMed]
- Kim IA, Bae SS, Fernandes A, et al. Selective inhibition of Ras, phosphoinositide 3 kinase, and Akt isoforms increases the radiosensitivity of human carcinoma cell lines. Cancer Res 2005;65:7902-10. [PubMed]
- Minjgee M, Toulany M, Kehlbach R, et al. K-RAS(V12) induces autocrine production of EGFR ligands and mediates radioresistance through EGFR-dependent Akt signaling and activation of DNA-PKcs. Int J Radiat Oncol Biol Phys 2011;81:1506-14. [PubMed]
- Toulany M, Dittmann K, Kruger M, et al. Radioresistance of K-Ras mutated human tumor cells is mediated through EGFR-dependent activation of PI3K-AKT pathway. Radiother Oncol 2005;76:143-50. [PubMed]
- Leslie NR, Downes CP. PTEN function: how normal cells control it and tumour cells lose it. Biochem J 2004;382:1-11. [PubMed]
- Courtney KD, Corcoran RB, Engelman JA. The PI3K pathway as drug target in human cancer. J Clin Oncol 2010;28:1075-83. [PubMed]
- Sadeq V, Isar N, Manoochehr T. Association of sporadic breast cancer with PTEN/MMAC1/TEP1 promoter hypermethylation. Med Oncol 2011;28:420-3. [PubMed]
- Kurose K, Zhou XP, Araki T, et al. Frequent loss of PTEN expression is linked to elevated phosphorylated Akt levels, but not associated with p27 and cyclin D1 expression, in primary epithelial ovarian carcinomas. Am J Pathol 2001;158:2097-106. [PubMed]
- Bettstetter M, Berezowska S, Keller G, et al. Epidermal growth factor receptor, phosphatidylinositol-3-kinase catalytic subunit/PTEN, and KRAS/NRAS/BRAF in primary resected esophageal adenocarcinomas: loss of PTEN is associated with worse clinical outcome. Hum Pathol 2013;44:829-36. [PubMed]
- Altomare DA, Tanno S, De Rienzo A, et al. Frequent activation of AKT2 kinase in human pancreatic carcinomas. J Cell Biochem 2002;87:470-6. [PubMed]
- Lim J, Kim JH, Paeng JY, et al. Prognostic value of activated Akt expression in oral squamous cell carcinoma. J Clin Pathol 2005;58:1199-205. [PubMed]
- Dobashi Y, Kimura M, Matsubara H, et al. Molecular alterations in AKT and its protein activation in human lung carcinomas. Hum Pathol 2012;43:2229-40. [PubMed]
- Ringel MD, Hayre N, Saito J, et al. Overexpression and overactivation of Akt in thyroid carcinoma. Cancer Res 2001;61:6105-11. [PubMed]
- Staal SP. Molecular cloning of the akt oncogene and its human homologues AKT1 and AKT2: amplification of AKT1 in a primary human gastric adenocarcinoma. Proc Natl Acad Sci U S A 1987;84:5034-7. [PubMed]
- Kirkegaard T, Witton CJ, Edwards J, et al. Molecular alterations in AKT1, AKT2 and AKT3 detected in breast and prostatic cancer by FISH. Histopathology 2010;56:203-11. [PubMed]
- Cohen Y, Shalmon B, Korach J, et al. AKT1 pleckstrin homology domain E17K activating mutation in endometrial carcinoma. Gynecol Oncol 2010;116:88-91. [PubMed]
- Askham JM, Platt F, Chambers PA, et al. AKT1 mutations in bladder cancer: identification of a novel oncogenic mutation that can cooperate with E17K. Oncogene 2010;29:150-5. [PubMed]
- Malanga D, Scrima M, De Marco C, et al. Activating E17K mutation in the gene encoding the protein kinase AKT1 in a subset of squamous cell carcinoma of the lung. Cell Cycle 2008;7:665-9. [PubMed]
- Bleeker FE, Felicioni L, Buttitta F, et al. AKT1(E17K) in human solid tumours. Oncogene 2008;27:5648-50. [PubMed]
- Yi KH, Axtmayer J, Gustin JP, et al. Functional analysis of non-hotspot AKT1 mutants found in human breast cancers identifies novel driver mutations: implications for personalized medicine. Oncotarget 2013;4:29-34. [PubMed]
- Hill MM, Hemmings BA. Inhibition of protein kinase B/Akt. implications for cancer therapy. Pharmacol Ther 2002;93:243-51. [PubMed]
- Cheng JQ, Ruggeri B, Klein WM, et al. Amplification of AKT2 in human pancreatic cells and inhibition of AKT2 expression and tumorigenicity by antisense RNA. Proc Natl Acad Sci U S A 1996;93:3636-41. [PubMed]
- Ottenhof NA, de Wilde RF, Maitra A, et al. Molecular characteristics of pancreatic ductal adenocarcinoma. Patholog Res Int 2011;2011:620601.
- Xu X, Sakon M, Nagano H, et al. Akt2 expression correlates with prognosis of human hepatocellular carcinoma. Oncol Rep 2004;11:25-32. [PubMed]
- Nakayama K, Nakayama N, Kurman RJ, et al. Sequence mutations and amplification of PIK3CA and AKT2 genes in purified ovarian serous neoplasms. Cancer Biol Ther 2006;5:779-85. [PubMed]
- Bacus SS, Altomare DA, Lyass L, et al. AKT2 is frequently upregulated in HER-2/neu-positive breast cancers and may contribute to tumor aggressiveness by enhancing cell survival. Oncogene 2002;21:3532-40. [PubMed]
- Cheng JQ, Godwin AK, Bellacosa A, et al. AKT2, a putative oncogene encoding a member of a subfamily of protein-serine/threonine kinases, is amplified in human ovarian carcinomas. Proc Natl Acad Sci U S A 1992;89:9267-71. [PubMed]
- Bellacosa A, de Feo D, Godwin AK, et al. Molecular alterations of the AKT2 oncogene in ovarian and breast carcinomas. Int J Cancer 1995;64:280-5. [PubMed]
- Liu AX, Testa JR, Hamilton TC, et al. AKT2, a member of the protein kinase B family, is activated by growth factors, v-Ha-ras, and v-src through phosphatidylinositol 3-kinase in human ovarian epithelial cancer cells. Cancer Res 1998;58:2973-7. [PubMed]
- Soung YH, Lee JW, Nam SW, et al. Mutational analysis of AKT1, AKT2 and AKT3 genes in common human carcinomas. Oncology 2006;70:285-9. [PubMed]
- Cristiano BE, Chan JC, Hannan KM, et al. A specific role for AKT3 in the genesis of ovarian cancer through modulation of G(2)-M phase transition. Cancer Res 2006;66:11718-25. [PubMed]
- Nakatani K, Thompson DA, Barthel A, et al. Up-regulation of Akt3 in estrogen receptor-deficient breast cancers and androgen-independent prostate cancer lines. J Biol Chem 1999;274:21528-32. [PubMed]
- Stahl JM, Sharma A, Cheung M, et al. Deregulated Akt3 activity promotes development of malignant melanoma. Cancer Res 2004;64:7002-10. [PubMed]
- Davies MA, Stemke-Hale K, Tellez C, et al. A novel AKT3 mutation in melanoma tumours and cell lines. Br J Cancer 2008;99:1265-8. [PubMed]
- Masure S, Haefner B, Wesselink JJ, et al. Molecular cloning, expression and characterization of the human serine/threonine kinase Akt-3. Eur J Biochem 1999;265:353-60. [PubMed]
- Madhunapantula SV, Sharma A, Robertson GP. PRAS40 deregulates apoptosis in malignant melanoma. Cancer Res 2007;67:3626-36. [PubMed]
- Nijkamp MM, Hoogsteen IJ, Span PN, et al. Spatial relationship of phosphorylated epidermal growth factor receptor and activated AKT in head and neck squamous cell carcinoma. Radiother Oncol 2011;101:165-70. [PubMed]
- Gazdar AF, Minna JD. Deregulated EGFR signaling during lung cancer progression: mutations, amplicons, and autocrine loops. Cancer Prev Res (Phila) 2008;1:156-60. [PubMed]
- Gazdar AF, Minna JD. Inhibition of EGFR signaling: all mutations are not created equal. PLoS Med 2005;2:e377 [PubMed]
- Kasten-Pisula U, Saker J, Eicheler W, et al. Cellular and tumor radiosensitivity is correlated to epidermal growth factor receptor protein expression level in tumors without EGFR amplification. Int J Radiat Oncol Biol Phys 2011;80:1181-8. [PubMed]
- Sheridan MT, O’Dwyer T, Seymour CB, et al. Potential indicators of radiosensitivity in squamous cell carcinoma of the head and neck. Radiat Oncol Investig 1997;5:180-6. [PubMed]
- Dittmann K, Mayer C, Fehrenbacher B, et al. Nuclear epidermal growth factor receptor modulates cellular radio-sensitivity by regulation of chromatin access. Radiother Oncol 2011;99:317-22. [PubMed]
- Huber SM, Misovic M, Mayer C, et al. EGFR-mediated stimulation of sodium/glucose cotransport promotes survival of irradiated human A549 lung adenocarcinoma cells. Radiother Oncol 2012;103:373-9. [PubMed]
- Toulany M, Dittmann K, Baumann M, et al. Radiosensitization of Ras-mutated human tumor cells in vitro by the specific EGF receptor antagonist BIBX1382BS. Radiother Oncol 2005;74:117-29. [PubMed]
- Zhang T, Cui GB, Zhang J, et al. Inhibition of PI3 kinases enhances the sensitivity of non-small cell lung cancer cells to ionizing radiation. Oncol Rep 2010;24:1683-9. [PubMed]
- Valerie K, Yacoub A, Hagan MP, et al. Radiation-induced cell signaling: inside-out and outside-in. Mol Cancer Ther 2007;6:789-801. [PubMed]
- Toulany M, Minjgee M, Kehlbach R, et al. ErbB2 expression through heterodimerization with erbB1 is necessary for ionizing radiation- but not EGF-induced activation of Akt survival pathway. Radiother Oncol 2010;97:338-45. [PubMed]
- Li HF, Kim JS, Waldman T. Radiation-induced Akt activation modulates radioresistance in human glioblastoma cells. Radiat Oncol 2009;4:43. [PubMed]
- Bussink J, van der Kogel AJ, Kaanders JH. Activation of the PI3-K/AKT pathway and implications for radioresistance mechanisms in head and neck cancer. Lancet Oncol 2008;9:288-96. [PubMed]
- Zhan M, Han ZC. Phosphatidylinositide 3-kinase/AKT in radiation responses. Histol Histopathol 2004;19:915-23. [PubMed]
- Price BD, Youmell MB. The phosphatidylinositol 3-kinase inhibitor wortmannin sensitizes murine fibroblasts and human tumor cells to radiation and blocks induction of p53 following DNA damage. Cancer Res 1996;56:246-50. [PubMed]
- Liu Y, Cui B, Qiao Y, et al. Phosphoinositide-3-kinase inhibition enhances radiosensitization of cervical cancer in vivo. Int J Gynecol Cancer 2011;21:100-5. [PubMed]
- Brognard J, Clark AS, Ni Y, et al. Akt/protein kinase B is constitutively active in non-small cell lung cancer cells and promotes cellular survival and resistance to chemotherapy and radiation. Cancer Res 2001;61:3986-97. [PubMed]
- Prevo R, Deutsch E, Sampson O, et al. Class I PI3 kinase inhibition by the pyridinyl-furanopyrimidine inhibitor PI-103 enhances tumor radiosensitivity. Cancer Res 2008;68:5915-23. [PubMed]
- Fuhrman CB, Kilgore J, LaCoursiere YD, et al. Radiosensitization of cervical cancer cells via double-strand DNA break repair inhibition. Gynecol Oncol 2008;110:93-8. [PubMed]
- Choi EJ, Ryu YK, Kim SY, et al. Targeting epidermal growth factor receptor-associated signaling pathways in non-small cell lung cancer cells: implication in radiation response. Mol Cancer Res 2010;8:1027-36. [PubMed]
- Lee CM, Fuhrman CB, Planelles V, et al. Phosphatidylinositol 3-kinase inhibition by LY294002 radiosensitizes human cervical cancer cell lines. Clin Cancer Res 2006;12:250-6. [PubMed]
- Tanno S, Yanagawa N, Habiro A, et al. Serine/threonine kinase AKT is frequently activated in human bile duct cancer and is associated with increased radioresistance. Cancer Res 2004;64:3486-90. [PubMed]
- Gupta AK, McKenna WG, Weber CN, et al. Local recurrence in head and neck cancer: relationship to radiation resistance and signal transduction. Clin Cancer Res 2002;8:885-92. [PubMed]
- Kim TJ, Lee JW, Song SY, et al. Increased expression of pAKT is associated with radiation resistance in cervical cancer. Br J Cancer 2006;94:1678-82. [PubMed]
- Datta SR, Dudek H, Tao X, et al. Akt phosphorylation of BAD couples survival signals to the cell-intrinsic death machinery. Cell 1997;91:231-41. [PubMed]
- Mitsiades CS, Mitsiades N, Poulaki V, et al. Activation of NF-kappaB and upregulation of intracellular anti-apoptotic proteins via the IGF-1/Akt signaling in human multiple myeloma cells: therapeutic implications. Oncogene 2002;21:5673-83. [PubMed]
- Martelli AM, Tazzari PL, Tabellini G, et al. A new selective AKT pharmacological inhibitor reduces resistance to chemotherapeutic drugs, TRAIL, all-trans-retinoic acid, and ionizing radiation of human leukemia cells. Leukemia 2003;17:1794-805. [PubMed]
- Choi SY, Kim MJ, Kang CM, et al. Activation of Bak and Bax through c-abl-protein kinase Cdelta-p38 MAPK signaling in response to ionizing radiation in human non-small cell lung cancer cells. J Biol Chem 2006;281:7049-59. [PubMed]
- Gardai SJ, Hildeman DA, Frankel SK, et al. Phosphorylation of Bax Ser184 by Akt regulates its activity and apoptosis in neutrophils. J Biol Chem 2004;279:21085-95. [PubMed]
- Toulany M, Kehlbach R, Florczak U, et al. Targeting of AKT1 enhances radiation toxicity of human tumor cells by inhibiting DNA-PKcs-dependent DNA double-strand break repair. Mol Cancer Ther 2008;7:1772-81. [PubMed]
- Hambardzumyan D, Becher OJ, Rosenblum MK, et al. PI3K pathway regulates survival of cancer stem cells residing in the perivascular niche following radiation in medulloblastoma in vivo. Genes Dev 2008;22:436-48. [PubMed]
- Baumann M, Krause M, Hill R. Exploring the role of cancer stem cells in radioresistance. Nat Rev Cancer 2008;8:545-54. [PubMed]
- Zhan JF, Wu LP, Chen LH, et al. Pharmacological inhibition of AKT sensitizes MCF-7 human breast cancer-initiating cells to radiation. Cell Oncol (Dordr) 2011;34:451-6. [PubMed]
- Zhang M, Atkinson RL, Rosen JM. Selective targeting of radiation-resistant tumor-initiating cells. Proc Natl Acad Sci U S A 2010;107:3522-7. [PubMed]
- Singh S, Trevino J, Bora-Singhal N, et al. EGFR/Src/Akt signaling modulates Sox2 expression and self-renewal of stem-like side-population cells in non-small cell lung cancer. Mol Cancer 2012;11:73. [PubMed]
- Mihatsch J, Toulany M, Bareiss PM, et al. Selection of radioresistant tumor cells and presence of ALDH1 activity in vitro. Radiother Oncol 2011;99:300-6. [PubMed]
- Apel A, Herr I, Schwarz H, et al. Blocked autophagy sensitizes resistant carcinoma cells to radiation therapy. Cancer Res 2008;68:1485-94. [PubMed]
- Rouschop KM, van den Beucken T, Dubois L, et al. The unfolded protein response protects human tumor cells during hypoxia through regulation of the autophagy genes MAP1LC3B and ATG5. J Clin Invest 2010;120:127-41. [PubMed]
- Chaachouay H, Ohneseit P, Toulany M, et al. Autophagy contributes to resistance of tumor cells to ionizing radiation. Radiother Oncol 2011;99:287-92. [PubMed]
- Fujiwara K, Iwado E, Mills GB, et al. Akt inhibitor shows anticancer and radiosensitizing effects in malignant glioma cells by inducing autophagy. Int J Oncol 2007;31:753-60. [PubMed]
- Toulany M, Kasten-Pisula U, Brammer I, et al. Blockage of epidermal growth factor receptor-phosphatidylinositol 3-kinase-AKT signaling increases radiosensitivity of K-RAS mutated human tumor cells in vitro by affecting DNA repair. Clin Cancer Res 2006;12:4119-26. [PubMed]
- Raju U, Riesterer O, Wang ZQ, et al. Dasatinib, a multi-kinase inhibitor increased radiation sensitivity by interfering with nuclear localization of epidermal growth factor receptor and by blocking DNA repair pathways. Radiother Oncol 2012;105:241-9. [PubMed]
- Myllynen L, Rieckmann T, Dahm-Daphi J, et al. In tumor cells regulation of DNA double strand break repair through EGF receptor involves both NHEJ and HR and is independent of p53 and K-Ras status. Radiother Oncol 2011;101:147-51. [PubMed]
- Toulany M, Lee KJ, Fattah KR, et al. Akt1 promotes post-irradiation survival of human tumor cells through initiation, progression and termination of DNA-PKcs-dependent DNA-double strand break repair. Mol Cancer Res 2012;10:945-57. [PubMed]
- Fraser M, Harding SM, Zhao H, et al. MRE11 promotes AKT phosphorylation in direct response to DNA double-strand breaks. Cell cycle 2011;10:2218-32. [PubMed]
- Khanna KK, Jackson SP. DNA double-strand breaks: signaling, repair and the cancer connection. Nat Genet 2001;27:247-54. [PubMed]
- Iliakis G, Wang H, Perrault AR, et al. Mechanisms of DNA double strand break repair and chromosome aberration formation. Cytogenet Genome Res 2004;104:14-20. [PubMed]
- Hsu FM, Zhang S, Chen BP. Role of DNA-dependent protein kinase catalytic subunit in cancer development and treatment. Transl Cancer Res 2012;1:22-34. [PubMed]
- Bouchaert P, Guerif S, Debiais C, et al. DNA-PKcs expression predicts response to radiotherapy in prostate cancer. Int J Radiat Oncol Biol Phys 2012;84:1179-85. [PubMed]
- Povirk LF, Zhou RZ, Ramsden DA, et al. Phosphorylation in the serine/threonine 2609-2647 cluster promotes but is not essential for DNA-dependent protein kinase-mediated nonhomologous end joining in human whole-cell extracts. Nucleic Acids Res 2007;35:3869-78. [PubMed]
- Douglas P, Sapkota GP, Morrice N, et al. Identification of in vitro and in vivo phosphorylation sites in the catalytic subunit of the DNA-dependent protein kinase. Biochem J 2002;368:243-51. [PubMed]
- Chen BP, Chan DW, Kobayashi J, et al. Cell cycle dependence of DNA-dependent protein kinase phosphorylation in response to DNA double strand breaks. J Biol Chem 2005;280:14709-15. [PubMed]
- Chen BP, Uematsu N, Kobayashi J, et al. Ataxia telangiectasia mutated (ATM) is essential for DNA-PKcs phosphorylations at the Thr-2609 cluster upon DNA double strand break. J Biol Chem 2007;282:6582-7. [PubMed]
- Park J, Feng J, Li Y, et al. DNA-dependent protein kinase-mediated phosphorylation of protein kinase B requires a specific recognition sequence in the C-terminal hydro-phobic motif. J Biol Chem 2009;284:6169-74. [PubMed]
- Bozulic L, Surucu B, Hynx D, et al. PKBalpha/Akt1 acts downstream of DNA-PK in the DNA double-strand break response and promotes survival. Mol Cell 2008;30:203-13. [PubMed]
- Toulany M, Schickfluss TA, Fattah KR, et al. Function of erbB receptors and DNA-PKcs on phosphorylation of cytoplasmic and nuclear Akt at S473 induced by erbB1 ligand and ionizing radiation. Radiother Oncol 2011;101:140-6. [PubMed]
- Mukherjee B, Choy H, Nirodi C, et al. Targeting nonhomologous end-joining through epidermal growth factor receptor inhibition: rationale and strategies for radiosensitization. Semin Radiat Oncol 2010;20:250-7. [PubMed]
- Meyn RE. Linking PTEN with genomic instability and DNA repair. Cell Cycle 2009;8:2322-3. [PubMed]
- Affolter A, Drigotas M, Fruth K, et al. Increased radioresistance via G12S K-Ras by compensatory upregulation of MAPK and PI3K pathways in epithelial cancer. Head Neck 2013;35:220-8. [PubMed]
- Lavin MF. ATM and the Mre11 complex combine to recognize and signal DNA double-strand breaks. Oncogene 2007;26:7749-58. [PubMed]
- Jeggo PA, Geuting V, Lobrich M. The role of homologous recombination in radiation-induced double-strand break repair. Radiother Oncol 2011;101:7-12. [PubMed]
- Goodarzi AA, Jeggo P, Lobrich M. The influence of heterochromatin on DNA double strand break repair: Getting the strong, silent type to relax. DNA repair 2010;9:1273-82. [PubMed]
- Beucher A, Birraux J, Tchouandong L, et al. ATM and Artemis promote homologous recombination of radiation-induced DNA double-strand breaks in G2. EMBO J 2009;28:3413-27. [PubMed]
- Viniegra JG, Martinez N, Modirassari P, et al. Full activation of PKB/Akt in response to insulin or ionizing radiation is mediated through ATM. The Journal of biological chemistry 2005;280:4029-36. [PubMed]
- Viniegra JG, Martinez N, Modirassari P, et al. Full activation of PKB/Akt in response to insulin or ionizing radiation is mediated through ATM. J Biol Chem 2005;280:4029-36. [PubMed]
- Khalil A, Morgan RN, Adams BR, et al. ATM-dependent ERK signaling via AKT in response to DNA double-strand breaks. Cell Cycle 2011;10:481-91. [PubMed]
- Hawkins AJ, Golding SE, Khalil A, et al. DNA double-strand break - induced pro-survival signaling. Radiother Oncol 2011;101:13-7. [PubMed]
- Brandsma I, Gent DC. Pathway choice in DNA double strand break repair: observations of a balancing act. Genome Integr 2012;3:9. [PubMed]
- Miki Y, Swensen J, Shattuck-Eidens D, et al. A strong candidate for the breast and ovarian cancer susceptibility gene BRCA1. Science 1994;266:66-71. [PubMed]
- Martin AM, Blackwood MA, Antin-Ozerkis D, et al. Germline mutations in BRCA1 and BRCA2 in breast-ovarian families from a breast cancer risk evaluation clinic. J Clin Oncol 2001;19:2247-53. [PubMed]
- Xiang T, Jia Y, Sherris D, et al. Targeting the Akt/mTOR pathway in Brca1-deficient cancers. Oncogene 2011;30:2443-50. [PubMed]
- Plo I, Laulier C, Gauthier L, et al. AKT1 inhibits homologous recombination by in-ducing cytoplasmic retention of BRCA1 and RAD51. Cancer Res 2008;68:9404-12. [PubMed]
- Jia Y, Song W, Zhang F, et al. Akt1 inhibits homologous recombination in Brca1-deficient cells by blocking the Chk1-Rad51 pathway. Oncogene 2013;32:1943-9. [PubMed]
- Puc J, Parsons R. PTEN loss inhibits CHK1 to cause double stranded-DNA breaks in cells. Cell Cycle 2005;4:927-9. [PubMed]
- Li L, Wang H, Yang ES, et al. Erlotinib attenuates homologous recombinational repair of chromosomal breaks in human breast cancer cells. Cancer Res 2008;68:9141-6. [PubMed]
- Konstantinidou G, Bey EA, Rabellino A, et al. Dual phosphoinositide 3-kinase/mammalian target of rapamycin blockade is an effective radiosensitizing strategy for the treatment of non-small cell lung cancer harboring K-RAS mutations. Cancer Res 2009;69:7644-52. [PubMed]
- Narayan RS, Fedrigo CA, Stalpers LJ, et al. Targeting the Akt-pathway to improve radiosensitivity in glioblastoma. Curr Pharm Des 2013;19:951-7. [PubMed]