Changes of tryptase in patients with hepatocellular carcinoma after transarterial chemoembolization
Introduction
Hepatocellular carcinoma (HCC) is one of the most common malignant tumors in the world (1). Because of the typically advanced nature of the occult disease at the time of diagnosis, the majority of patients with HCC have no chance to accept surgical resection. Transarterial chemoembolization (TACE) can improve the quality of life and survival time of patients with HCC (2-7). At present, TACE has become the main therapeutic method for patients with HCC without the option for surgical resection. However, tumor angiogenesis of residual lesions seriously affects the efficacy of TACE in the locoregional treatment of HCC (8-11). A variety of studies have demonstrated the classical regulatory factors and the vital inducing factors involved in all stages of tumor angiogenesis; changes in the expression of these factors after TACE treatment for HCC were also observed (8-14). In recent years, a few studies have shown that tryptase released by mast cells (MCs) correlates with tumor angiogenesis in several types of malignancies (15-27); however, few studies have addressed the dynamic changes of tryptase after TACE and the use of monitoring the treatment response to TACE in HCC patients. Therefore, the present study aimed to detect the serum tryptase levels in patients with HCC 1 day before and 1, 7 and 28 days after TACE to explore the dynamic changes of tryptase in HCC after TACE treatment and to determine whether the tryptase level changes in response to TACE.
Methods
Clinical data
During the period from October 2013 to November 2014, a total of 40 HCC patients were selected, including 36 males and 4 females. All cases were confirmed by percutaneous liver biopsy or typical imaging findings (dynamic enhanced computed tomography or magnetic resonance imaging) for HCC associated with a pathological increase of serum alpha-fetoprotein levels. The ages of the patients ranged from 35 to 70 years (mean age 56.3±9.9 years). None of the patients received any other anti-tumor therapy prior to the TACE procedure. Four weeks after chemoembolization procedure, the overall tumor response was evaluated according to the modified Response Evaluation Criteria in Solid Tumors (mRECIST) (28).
TACE procedure
In 40 patients, the chemoembolization procedure was conducted according to the method reported in our previous study (29). A 5-F RH angiographic catheter (Terumo Corporation, 44-1, 2-Chome, Hatagaya, Shibuya-ku, Tokyo 151-0072, Japan) was placed in the common or proper hepatic artery for diagnostic arteriography. Following the superselective catheterization procedure with a microcatheter (3F SP microcatheter, Terumo Corporation, 44-1, 2-Chome, Hatagaya, Shibuya-ku, Tokyo 151-0072, Japan), TACE was performed by the administration of 5-fluorouracil (1,000 mg) and hydroxycamptothecine (30 mg), followed by lipiodol with adriamycin (50 mg) emulsion and gelfoam particles.
Experimental methods
Fasting peripheral venous blood samples (3–5 mL) were collected from each patient in the morning 1 day prior to the TACE procedure, as well as 1, 7, and 28 days after TACE treatment. All of the samples were placed in sterile tubes and were allowed to stand for 30–60 min. Then, the samples were centrifuged at 3,000 r/min for 15 min; the serum samples were collected and stored in a −80 °C freezer for future use. Tryptase levels were determined using enzyme-linked immunosorbent assays (ELISAs) according to the ELISA kit instructions.
Statistical methods
SPSS 20.0 was used to conduct the statistical analysis. The level of serum tryptase was expressed as the mean ± standard deviation. The difference in serum tryptase levels before and after chemoembolization therapy was examined using analysis of variance. A t-test was performed for comparisons between two response groups, P<0.05 was considered statistically significant.
Results
Liver function in HCC patients was evaluated using the Child-Pugh grading scale. Twenty-nine patients (72.5%) were classified as Child-Pugh grade A, while 11 patients (27.5%) were classified as Child-Pugh grade B. The levels of tryptase in this group 1 day before TACE, and 1, 7 and 28 days after TACE were 4.85±2.46, 3.32±1.76, 3.15±1.67 and 4.85±2.50 ng/mL, respectively. The tryptase levels before and after TACE treatment were significantly different (F=48.461, P=0.000) (Figure 1). The levels of tryptase decreased markedly on day 1 and 7 after TACE and were recovered to the pre-TACE level on day 28 after TACE. Partial response (PR), stable disease (SD) and progressive disease (PD) were observed in 13 (32.5%), 12 (30.0%) and 15 (37.5%) patients, respectively; none of the patients achieved a complete response (CR). Patients with CR + PR constituted the responsive group, and patients with SD + PD constituted the non-responsive group. The serum levels of tryptase in responsive group were significantly lower than those in non-responsive group 28 days after TACE (3.54±1.16 vs. 5.48±2.74 ng/mL, respectively; t=2.426, P=0.020) (Figure 2).
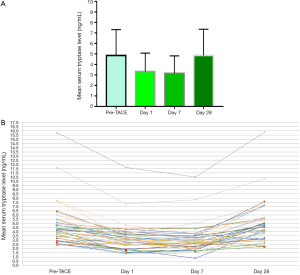
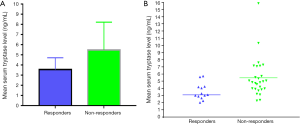
Discussion
The normal liver receives a dual supply of blood from both the portal vein and the hepatic artery. The portal vein is responsible for 80% of the blood supply to normal liver tissue. In contrast, the blood supply to hepatic tumors is mainly delivered by the hepatic artery. TACE involves both anti-cancer chemotherapy and embolization of tumor-feeding arteries. Recently, the Barcelona Clinic Liver Cancer (BCLC) classification emerged as the standard classification system for the clinical management of patients with HCC. According to this staging system, TACE is recommended as standard therapy for patients with intermediate-stage HCC (B stage of BCLC staging criteria) (4,30,31).
Previous research has shown that MCs are present in both normal and pathological livers (32-36). MC density is highly correlated with angiogenesis in chronic inflammatory diseases and tumors (37-41). Human MCs are categorized into those positive only for tryptase and those positive for both tryptase and chymase, and tryptase appears to play a more significant role in tumor progression than chymase (42). However, the role of tryptase in tumor angiogenesis is not entirely clear. Previous studies have shown that tryptase may induce tumor angiogenesis through the following mechanisms (42-48). First, tryptase interacts directly with endothelial cells via an unidentified mechanism to induce angiogenesis, and second, tryptase activates latent metalloproteinases and plasminogen activator to degrade the extracellular matrix, and this degradation is critical in the early stages of angiogenesis.
The process of tumor angiogenesis is very complex. There are many factors involved in tumor angiogenesis. Vascular endothelial growth factor (VEGF), a classical angiogenesis stimulating factor, plays a key role in tumor angiogenesis (8). Bai et al. (49) found that addition of tryptase to human dermal microvascular endothelial cells (HDMECs) caused an obvious increase of mRNA and protein levels of VEGF and its receptors. Yang et al. (50) investigated the correlation between beta-tryptase and VEGF in bone marrow stromal cells in acute myeloid leukemia. They found that VEGF mRNA and protein expression was significantly up-regulated by beta-tryptase in a dose-dependent manner. These results suggest that tryptase significantly increased the expressions of VEGF. However, Ranieri et al. (51) evaluated serum levels of both VEGF and tryptase in 71 HCC patients. Their results demonstrated the lack of correlation between serum VEGF and tryptase levels suggesting an independent role of VEGF and tryptase in the angiogenic process.
Goffredo et al. (52) reported a study about the potential significance of serum tryptase in HCC patients in 2013. These authors assessed serum tryptase levels in 30 HCC patients 1 day before, and 1 day after TACE. In their study, a statistically significant difference was detected between pre- and post-TACE tryptase levels. Ranieri et al. (51) reported similar results. These results demonstrated that tryptase was a potential biomarker of the response to TACE treatment in HCC patients. In the present study, the serum tryptase levels decreased significantly 1 day after TACE treatment compared with those measured before TACE treatment, and the levels remained low at 7 days after TACE; however, the serum levels of tryptase in this group returned to pre-TACE levels at 28 days after TACE. Moreover, the tryptase levels in different response groups 28 days after TACE treatment were significantly different. The change in tryptase level observed 1 day after TACE was consistent with the results of Goffredo et al. (52) and Ranieri et al. (51). One possible reason for why the tryptase level decreased significantly 1 day and 7 days after TACE treatment may be that TACE causes tumor tissue ischemia and necrosis, leading to decreased numbers of MCs and less secretion of tryptase. However, TACE also causes local hypoxia and stimulates angiogenesis via VEGF expression that in turns may help residual cancer cells to survival. Thus, serum tryptase may be a surrogate indicator of the magnitude of the angiogenic process and of the presence of HCC tumor tissues (51). It may be used to evaluate therapeutic responses to TACE in combination of other serum biomarkers as well as imaging biomarkers (4,53-58).
However, the present study has several limitations. First, the number of cases was small. Second, the serum tryptase levels in patients with HCC after TACE are known to change dynamically at different times; however, in this study, the serum levels of tryptase were detected only at limited time points. Third, the relationship between tryptase and other classical tumor angiogenesis regulatory factors, such as VEGF, was not studied.
In summary, the level of serum tryptase in patients with HCC after TACE shows a dynamic change that may be used to evaluate the response to TACE.
Acknowledgments
Funding: This work was supported by the projects of department of Science and Technology of Sichuan Province (2016JY0105).
Footnote
Provenance and Peer Review: This article was commissioned by the Guest Editors (Yì-Xiáng J. Wáng, Yong Wang) for the series “Translational Imaging in Cancer Patient Care” published in Translational Cancer Research. The article has undergone external peer review.
Conflicts of Interest: All authors have completed the ICMJE uniform disclosure form (available at http://dx.doi.org/10.21037/tcr.2017.08.43). The series “Translational Imaging in Cancer Patient Care” was commissioned by the editorial office without any funding or sponsorship. The authors have no other conflicts of interest to declare.
Ethical Statement: The authors are accountable for all aspects of the work in ensuring that questions related to the accuracy or integrity of any part of the work are appropriately investigated and resolved. The study was conducted in accordance with the Declaration of Helsinki (as revised in 2013). This study was approved by our institutional review board, and patient informed consent (written consent) was obtained.
Open Access Statement: This is an Open Access article distributed in accordance with the Creative Commons Attribution-NonCommercial-NoDerivs 4.0 International License (CC BY-NC-ND 4.0), which permits the non-commercial replication and distribution of the article with the strict proviso that no changes or edits are made and the original work is properly cited (including links to both the formal publication through the relevant DOI and the license). See: https://creativecommons.org/licenses/by-nc-nd/4.0/.
References
- Ferlay J, Shin HR, Bray F, et al. Estimates of worldwide burden of cancer in 2008: GLOBOCAN 2008. Int J Cancer 2010;127:2893-917. [Crossref] [PubMed]
- Lo CM, Ngan H, Tso WK, et al. Randomized controlled trial of transarterial lipiodol chemoembolization for unresectable hepatocellular carcinoma. Hepatology 2002;35:1164-71. [Crossref] [PubMed]
- Llovet JM, Real MI, Montaña X, et al. Arterial embolisation or chemoembolisation versus symptomatic treatment in patients with unresectable hepatocellular carcinoma: a randomised controlled trial. Lancet 2002;359:1734-9. [Crossref] [PubMed]
- Wáng YX, De Baere T, Idée JM, et al. Transcatheter embolization therapy in liver cancer: an update of clinical evidences. Chin J Cancer Res 2015;27:96-121. [PubMed]
- Han K, Kim JH. Transarterial chemoembolization in hepatocellular carcinoma treatment: Barcelona clinic liver cancer staging system. World J Gastroenterol 2015;21:10327-35. [Crossref] [PubMed]
- Cammà C, Schepis F, Orlando A, et al. Transarterial chemoembolization for unresectable hepatocellular carcinoma: meta-analysis of randomized controlled trials. Radiology 2002;224:47-54. [Crossref] [PubMed]
- Marelli L, Stigliano R, Triantos C, et al. Transarterial therapy for hepatocellular carcinoma: which technique is more effective? A systematic review of cohort and randomized studies. Cardiovasc Intervent Radiol 2007;30:6-25. [Crossref] [PubMed]
- Liu K, Min XL, Peng J, et al. The Changes of HIF-1α and VEGF Expression After TACE in Patients With Hepatocellular Carcinoma. J Clin Med Res 2016;8:297-302. [Crossref] [PubMed]
- Gupta S, Kobayashi S, Phongkitkarun S, et al. Effect of transcatheter hepatic arterial embolization on angiogenesis in an animal model. Invest Radiol 2006;41:516-21. [Crossref] [PubMed]
- Guo WJ, Li J, Chen Z, et al. Transient increased expression of VEGF and MMP-1 in a rat liver tumor model after hepatic arterial occlusion. Hepatogastroenterology 2004;51:381-6. [PubMed]
- Xue T, Feng W, Yu H, et al. Metastasis-Associated Protein 1 Is Involved in Angiogenesis after Transarterial Chemoembolization Treatment. Biomed Res Int 2017;6757898 [PubMed]
- Yancopoulos GD, Davis S, Gale NW, et al. Vascular-specific growth factors and blood vessel formation. Nature 2000;407:242-8. [Crossref] [PubMed]
- Yamaguchi R, Yano H, Nakashima Y, et al. Expression and localization of vascular endothelial growth factor receptors in human hepatocellular carcinoma and non-HCC tissues. Oncol Rep 2000;7:725-9. [PubMed]
- Jing Q, Jianyong L, Jiming Y, et al. Predictive value of recurrence for serum hypoxia inducible factor-1α C-reaction protein in hepatocellular carcinoma patients after transcatheter arterial chemoembolization. Indian J Cancer 2015;52:e105-6. [Crossref] [PubMed]
- Ranieri G, Ammendola M, Patruno R, et al. Tryptase-positive mast cells correlate with angiogenesis in early breast cancer patients. Int J Oncol 2009;35:115-20. [Crossref] [PubMed]
- Ammendola M, Sacco R, Zuccalà V, et al. Mast Cells Density Positive to Tryptase Correlate with Microvascular Density in both Primary Gastric Cancer Tissue and Loco-Regional Lymph Node Metastases from Patients That Have Undergone Radical Surgery. Int J Mol Sci 2016;17. [PubMed]
- Micu GV, Stăniceanu F, Sticlaru LC, et al. Correlations Between the Density of Tryptase Positive Mast Cells (DMCT) and that of New Blood Vessels (CD105+) in Patients with Gastric Cancer. Rom J Intern Med 2016;54:113-20. [Crossref] [PubMed]
- Guo X, Zhai L, Xue R, et al. Mast Cell Tryptase Contributes to Pancreatic Cancer Growth through Promoting Angiogenesis via Activation of Angiopoietin-1. Int J Mol Sci 2016;17. [PubMed]
- Ammendola M, Patruno R, Sacco R, et al. Mast cells positive to tryptase and tumour-associated macrophages correlate with angiogenesis in locally advanced colorectal cancer patients undergone to surgery. Expert Opin Ther Targets 2016;20:533-40. [Crossref] [PubMed]
- Ammendola M, Sacco R, Sammarco G, et al. Mast cells positive to tryptase, endothelial cells positive to protease-activated receptor-2, and microvascular density correlate among themselves in hepatocellular carcinoma patients who have undergone surgery. Onco Targets Ther 2016;9:4465-71. [Crossref] [PubMed]
- Tamma R, Guidolin D, Annese T, et al. Spatial distribution of mast cells and macrophages around tumor glands in human breast ductal carcinoma. Exp Cell Res 2017;359:179-84. [Crossref] [PubMed]
- Chen Y, Li C, Xie H, et al. Infiltrating mast cells promote renal cell carcinoma angiogenesis by modulating PI3K→AKT→GSK3β→AM signaling. Oncogene 2017;36:2879-88. [Crossref] [PubMed]
- Sharma B, Sriram G, Saraswathi TR, et al. Immunohistochemical evaluation of mast cells and angiogenesis in oral squamous cell carcinoma. Indian J Dent Res 2010;21:260-5. [Crossref] [PubMed]
- Akbarzadeh Baghban A, Taghavi N, Shahla M. Combined Analysis of Vascular Endothelial Growth Factor Expression with Cyclooxygenase-2 and Mast Cell Density in Oral Squamous Cell Carcinoma. Pathobiology 2017;84:80-6. [Crossref] [PubMed]
- Guldur ME, Kocarslan S, Ozardali HI, et al. The relationship of mast cells and angiogenesis with prognosis in renal cell carcinoma. J Pak Med Assoc 2014;64:300-3. [PubMed]
- Vyzoukaki R, Tsirakis G, Pappa CA, et al. The Impact of Mast Cell Density on the Progression of Bone Disease in Multiple Myeloma Patients. Int Arch Allergy Immunol 2015;168:263-8. [Crossref] [PubMed]
- Cimpean AM, Tamma R, Ruggieri S, et al. Mast cells in breast cancer angiogenesis. Crit Rev Oncol Hematol 2017;115:23-6. [Crossref] [PubMed]
- Eisenhauer EA, Therasse P, Bogaerts J, et al. New response evaluation criteria in solid tumours: revised RECIST guideline (version 1.1). Eur J Cancer 2009;45:228-47. [Crossref] [PubMed]
- Yang L, Zhang XM, Tan BX, et al. Computed tomographic perfusion imaging for the therapeutic response of chemoembolization for hepatocellular carcinoma. J Comput Assist Tomogr 2012;36:226-30. [Crossref] [PubMed]
- European Association For The Study Of The Liver. EASL-EORTC clinical practice guidelines: management of hepatocellular carcinoma. J Hepatol 2012;56:908-43. [Crossref] [PubMed]
- Bruix J, Sherman MAmerican Association for the Study of Liver Diseases. Management of hepatocellular carcinoma: an update. Hepatology 2011;53:1020-2. [Crossref] [PubMed]
- Cervello M, Foderàa D, Florena AM, et al. Correlation between expression of cyclooxygenase-2 and the presence of inflammatory cells in human primary hepatocellular carcinoma: possible role in tumor promotion and angiogenesis. World J Gastroenterol 2005;11:4638-43. [Crossref] [PubMed]
- Ju MJ, Qiu SJ, Gao Q, et al. Combination of peritumoral mast cells and T-regulatory cells predicts prognosis of hepatocellular carcinoma. Cancer Sci 2009;100:1267-74. [Crossref] [PubMed]
- Peng SH, Deng H, Yang JF, et al. Significance and relationship between infiltrating inflammatory cell and tumor angiogenesis in hepatocellular carcinoma tissues. World J Gastroenterol 2005;11:6521-4. [Crossref] [PubMed]
- Terada T, Matsunaga Y. Increased mast cells in hepatocellular carcinoma and intrahepatic cholangiocarcinoma. J Hepatol 2000;33:961-6. [Crossref] [PubMed]
- Jarido V, Kennedy L, Hargrove L, et al. The emerging role of mast cells in liver disease. Am J Physiol Gastrointest Liver Physiol 2017;313:G89-G101. [Crossref] [PubMed]
- Norrby K. Mast cells and angiogenesis. APMIS 2002;110:355-71. [Crossref] [PubMed]
- Hagmann W, Hacker HJ, Buchholz U. Resident mast cells are the main initiators of anaphylactic leukotriene production in the liver. Hepatology 1992;16:1477-84. [Crossref] [PubMed]
- Ribatti D, Vacca A, Nico B, et al. Bone marrow angiogenesis and mast cell density increase simultaneously with progression of human multiple myeloma. Br J Cancer 1999;79:451-5. [Crossref] [PubMed]
- Sperr WR, El-Samahi A, Kundi M, et al. Elevated tryptase levels selectively cluster in myeloid neoplasms: a novel diagnostic approach and screen marker in clinical haematology. Eur J Clin Invest 2009;39:914-23. [Crossref] [PubMed]
- Marech I, Ammendola M, Sacco R, et al. Serum tryptase, mast cells positive to tryptase and microvascular density evaluation in early breast cancer patients: possible translational significance. BMC Cancer 2014;14:534. [Crossref] [PubMed]
- de Souza DA Junior, Santana AC, da Silva EZ, et al. The Role of Mast Cell Specific Chymases and Tryptases in Tumor Angiogenesis. Biomed Res Int 2015;2015:142359 [PubMed]
- Blair RJ, Meng H, Marchese MJ, et al. Human mast cells stimulate vascular tube formation. Tryptase is a novel, potent angiogenic factor. J Clin Invest 1997;99:2691-700. [Crossref] [PubMed]
- Hartmann T, Ruoss SJ, Raymond WW, et al. Human tryptase as a potent, cell-specific mitogen: role of signaling pathways in synergistic responses. Am J Physiol 1992;262:L528-34. [PubMed]
- Cairns JA, Walls AF. Mast cell tryptase is a mitogen for epithelial cells. Stimulation of IL-8 production and intercellular adhesion molecule-1 expression. J Immunol 1996;156:275-83. [PubMed]
- Ruoss SJ, Hartmann T, Caughey GH. Mast cell tryptase is a mitogen for cultured fibroblasts. J Clin Invest 1991;88:493-9. [Crossref] [PubMed]
- Frungieri MB, Albrecht M, Raemsch R, et al. The action of the mast cell product tryptase on cyclooxygenase-2 (COX2) and subsequent fibroblast proliferation involves activation of the extracellular signal-regulated kinase isoforms 1 and 2 (erk1/2). Cell Signal 2005;17:525-33. [Crossref] [PubMed]
- Kaminska R, Helisalmi P, Harvima RJ, et al. Focal dermal-epidermal separation and fibronectin cleavage in basement membrane by human mast cell tryptase. J Invest Dermatol 1999;113:567-73. [Crossref] [PubMed]
- Bai Q, Li X, Wang X, et al. VEGF is involved in the increase of dermal microvascular permeability induced by tryptase. ISRN Dermatol 2012;2012:941465 [PubMed]
- Yang XP, Li Y, Wang Y, et al. beta-Tryptase up-regulates vascular endothelial growth factor expression via proteinase-activated receptor-2 and mitogen-activated protein kinase pathways in bone marrow stromal cells in acute myeloid leukemia. Leuk Lymphoma 2010;51:1550-8. [Crossref] [PubMed]
- Ranieri G, Ammendola M, Marech I, et al. Vascular endothelial growth factor and tryptase changes after chemoembolization in hepatocarcinoma patients. World J Gastroenterol 2015;21:6018-25. [Crossref] [PubMed]
- Goffredo V, Gadaleta CD, Laterza A, et al. Tryptase serum levels in patients suffering from hepatocellular carcinoma undergoing intra-arterial chemoembolization: Possible predictive role of response to treatment. Mol Clin Oncol 2013;1:385-9. [Crossref] [PubMed]
- Mahmud MH, Nordin AJ, Ahmad Saad FF, et al. Impacts of biological and procedural factors on semiquantification uptake value of liver in fluorine-18 fluorodeoxyglucose positron emission tomography/computed tomography imaging. Quant Imaging Med Surg 2015;5:700-7. [PubMed]
- Liu K, Yang L, Zhang XM, et al. HIF-1α and VEGF levels for monitoring hepatocellular carcinoma treatment response to transcatheter arterial chemoembolization. Transl Cancer Res 2017;6:1043-9.
- Xu X, Luo L, Chen J, et al. Acoustic radiation force impulse elastography for efficacy evaluation after hepatocellular carcinoma radiofrequency ablation: a comparative study with contrast-enhanced ultrasound. Biomed Res Int 2014;2014:901642 [PubMed]
- Yuan J, Lo G, King AD. Functional magnetic resonance imaging techniques and their development for radiation therapy planning and monitoring in the head and neck cancers. Quant Imaging Med Surg 2016;6:430-48. [Crossref] [PubMed]
- Chilla GS, Tan CH, Xu C, et al. Diffusion weighted magnetic resonance imaging and its recent trend-a survey. Quant Imaging Med Surg 2015;5:407-22. [PubMed]
- Lewis HL, Ghasabeh MA, Khoshpouri P, et al. Functional hepatic imaging as a biomarker of primary and secondary tumor response to loco-regional therapies. Surg Oncol 2017;26:411-22. [Crossref] [PubMed]