FL118, a novel anticancer compound, inhibits proliferation and migration of ovarian cancer cells via up-regulation of cytoglobin in vivo and in vitro
Introduction
Camptothecins have great importance in anticancer compounds in 21st century due to their wide clinical applications (1). At present, irinotecan and topotecan are approved for cancer therapy by the Food and Drug Administration (FDA) (2,3). Unfortunately, an increasing number of irinotecan (4,5) and topotecan (6,7) resistance cases have been reported in the clinical application. Even worse, the hematopoietic toxicity and diarrhea have been considered as the major adverse effects of irinotecan and topotecan (8).
In an effort to overcoming resistance and reducing cytotoxicity during tumor chemotherapy, Dr. Fengzhi Li and his coworkers have identified a novel camptothecin derivative (designated as FL118) by targeting the human surviving gene via high throughput screening (HTS) of small molecule libraries (9). Although FL118 is similar to the structure of irinotecan or topotecan (9), the mechanism of drug action between FL118 and irinotecan/topotecan is completely different.
FL118 exhibits strong anticancer activity on colon, head-and-neck and non-small cell lung cancer (10), but little is known about FL118 in ovarian cancer, which is the leading cause of death among women compared with other gynecological malignant tumors (11,12). Cytoglobin (CYGB), located on chromosomal region 17q25, is usually lost in many malignancies (13). Increasing studies have reported that CYGB is closely related to many cancers, including oesophageal cancer (14,15), non-small lung cancer, breast cancer (16) and ovarian cancer (17). These results significantly indicate that CYGB functions as a tumor suppressor gene. Proteomic analysis in our previous research showed that the expression of CYGB was significantly increased after FL118 treatment. In this paper, the effects of FL118 in vitro and in vivo of ovarian cancer cells were investigated. It was found that FL118 significantly inhibited the cell proliferation and migration of ovarian cancer in a dose-dependent manner and exhibited better antitumor effect compared with topotecan, along with the up-regulation of CYGB expression, this might provide positive evidences supporting FL118’s clinical application in the future and represent an important molecular mechanism of FL118’s excellent antitumor effect.
Methods
Cell culture
A human ovarian cancer cell line, SK-O-V3, was obtained from Chinese Academy of Sciences. Another human ovarian carcinoma cell line, ES-2, was kindly donated from School of Pharmacy Qingdao University. The human colorectal cancer cell line, HCT116, was donated from Translational Medicine College of Qingdao University. Cells were grown either in DMEM/HIGH GLUCOSE (HyClone) medium (HCT116, ES-2) or in McCoy’s 5A (BOSTER) medium (SK-O-V3) with glutamine, which was supplemented with 10% fetal bovine serum (FBS; BIOTECH-PAN), along with 1% penicillin and streptomycin (HyClone) under a 5% CO2 humidified atm-osphere at 37 °C. Moreover, ES-2 was a human ovarian clear cell carcinoma, and SK-O-V3 was a human ovarian serous cystadenocarcinoma.
Chemical reagents
FL118 was supplied by American Roswell Park Cancer Institute (RPCI), which was our cooperating lab. Topotecan was purchased from Selleck (S1231, Shanghai, China).
Cell transfection
Infection of target cells with siRNA (GenePharma) was performed using Lipofectamine RNAIMAX (Invitrogen, CA, USA) according to the manufacturer’s protocols. Briefly, cells were seeded into a 6-well plate (2.0×/well) or a 96-well plate (1.5×/well) so that they would be 60–80% confluent at the time of transfection. The media were replaced with serum-free medium and the antibiotics had been omitted during transfection. Subsequently, 5 µL/1 µL siRNA and 5 µL/1 µL Lipofectamine RNAIMAX reagent was diluted in 100 µL/10 µL serum-free medium for 5 min respectively in 6-well plate or 96-well plate. Then diluted siRNA was added to the diluted Lipofectamine RNAIMAX reagent. This was followed by incubation for 20 min at room temperature and the siRNA-lipid complex was added to cells. Four hours after transfection, transfection mixture was removed and then the siRNA-infected or uninfected ovarian cancer cells were treated with/without FL118. MTT blocking assay was done after FL118 (100 nM) treatment for 48 hours and scratch wound blocking assay was done after FL118 (100 nM) treatment for 24 hours. Subsequently, cells were used for either MTT analysis or migration analysis. The related information about siRNA used in our study were the followings: sense 5'-AGAAAGUGCCAGGCGAGAUTT-3'and antisense 5'-AUCUCGCCUGGCACUUUCUTT-3' (CYGB-homo-368); sense 5'-GGAGUCCCUUAGGGAAUAATT-3' and antisense 5'-UUAUUCCCUAAGGGACUCCTT-3' (CYGB-homo-1237); sense 5'-AGACCCAGCUGUGUCAUUATT-3' and antisense 5'-UAAUGACACAGCUGGGUCUTT-3' (CYGB-homo-2119); sense 5'-UUCUCCGAACGUGUCACGUTT-3' and antisense 5'-ACGUGACACGUUCGGAGAATT-3' (Negative control). All experiments were performed three times. Moreover, SK-O-V3 was grown in McCoy’s 5A (BOSTER) medium without serum/antibiotics and ES-2 was cultured in DMEM/HIGH GLUCOSE (HyClone) medium without serum/antibiotics in the process of cell transfection.
Cell viability assay
Cells (7×103) were plated in 96-well plates. After 24 hours, cells were treated with FL118 at varying concentrations: 0/1/10/50/100/200 nM. After 24, 48 and 72 hours, 20 µL of 3-(4, 5-dimethyl-2-thiazolyl)-2, 5-diphenyl-2-H-tetrazolium bromide (MTT) (5 mg/mL) was added to 180 µL cells medium. This was followed by incubation for 4 hours, after which MTT was discarded and 200 µL of dimethyl sulfoxide (DMSO) was added. The OD value was measured on a microplate reader (Bio-Tek instruments) with 490 nm.
Scratch wound assay
Cancer cells (2×106) were plated in 6-well plates so that they would be 80–90% confluent overnight. Subsequently, a plastic 200 µL tip was used to create the scratch wound and remove the cell debris with PBS two times. Culture medium with 1% FBS was added to every well. Collectively, a series of concentrations of FL118 (10/100 nM) or medium was added and then cells were continuously cultured in an incubator at 37 °C for 24 hours. Micrographs of the migration of cells would be taken from three different sites at 0 and 24 hours using an inverted microscope (Olympus; magnification, 40×). Cell migration distance = distance at 0 hour − distance at 48 hours.
Quantitative real-time PCR
Total RNA was extracted from cells by Trizon reagent (ComWin Biotechnology Corporation, China). In turn, total RNA (1 µg per sample) was converted to cDNA with FastQuant RT Kit (TIANGEN, China) following the manufacturer’s protocols. Then corresponding cDNA was used for real-time qPCR with the SuperReal PreMix Plus. The primers used in our study were the following: 5’-CAGTTCAAGCACATGGAGGA-3’ (CYGB forward) and 5’-GTGGGAAGTCACTGGCAAAT-3’ (CYGB reverse); 5’-AAGAGAGGCATCCTGACCCT-3’ (β-actin forward) and 5’-TACATGGCTGGGGTGTTGAA-3’ (β-actin reverse). The real-time qPCR condition was 95 °C for 15 min, followed by 40 cycles at 95 °C for 10 sec, 55 °C for 20 sec and 72 °C 30 sec using 96-well PCR plates. At last, the data were analyzed by the Bio-Rad CFX Manager software and normalized to β-actin.
Immunoblotting assays
Total proteins of cultured cells were obtained with Protein Extraction Reagent (Solarbio, China), separated using 15% SDS-PAGE gel and transferred onto 0.45 µm polyvinyl difluoride (PVDF) membranes (Solarbio, China). The membranes were blocked with 5% skim milk for 1 hour in TBST and incubated with primary antibodies (1:1,000, listed below) at 4 °C. After overnight incubation, membranes were washed with TBST three times and then incubated with corresponding secondary antibodies (1:1,000) for 1.5 hours at room temperature. The primary antibodies involved in our work included: GAPDH (Abcam, ab181602), CYGB (Abcam, ab181380), E-cadherin (CST), N-cadherin (CST), Vimentin (CST), mTOR (CST), p-mTOR (CST), AKT (CST), and p-AKT (CST). The amount of proteins loaded on SDS-Page gels is 10 µg.
Animal experiments
The animal experimental procedures were followed according to the institutional and national guide for the use and care of laboratory animals. ES-2 cells (4×106) were injected subcutaneously into the left flanks of female BALB/c nude mice (7–8-week-old, 15–22 g, n=40). Animals were randomly divided into four groups: control (n=10), topotecan (2 mg/kg, n=10), low-dose FL118 (5 mg/kg, n=10), and high-dose FL118 (10 mg/kg, n=10) when tumors reached 100–200 mm3. The FL118 formulation and the corresponding vehicle solution formulation was prepared according to the basic method reported previously (18). Subsequently, FL118 was orally administered once a week for 20 days (19). Topotecan was administered five times a week for 20 days by oral gavage (2 mg/kg per administration) (20). Body weight and tumor volume were measured every other day during the treatment. The tumor volume was calculated with the following formula: V (mm3) = 1/2*(length × width2), and was periodically determined using calipers. When the volume ≥1,500 mm3 or a moribund condition, the mice were sacrificed and the expression of indicated proteins and RNA were detected in the dissected tumors by q RT-PCR or immunoblotting assay.
Statistical analysis
All the experiments and measurements were performed three times and all statistical analyses were performed by unpaired, two-tailed t-test. P<0.05 (*), P<0.01 (**) and P<0.001 (***) were considered to be statistically significant.
Results
FL118 inhibits the cell proliferation of ES-2 and SK-O-V3 cells in a time- and dose-dependent manner
The chemical structure of FL118 is shown in Figure 1A. In order to detect the inhibitory effect of FL118 on ovarian cancer cells, a series of FL118 doses (0, 1, 10, 50, 100 and 200 nM) were administrated in ES-2 and SK-O-V3 cells for 24, 48 and 72 hours. The inhibitory rate was markedly elevated with time after FL118 treatment and also significantly rose with the increasing concentration of FL118 (Figure 1B,C). Interestingly, ES-2 cells represented a higher sensitivity to FL118 compared to SK-O-V3 cells. The above data indicated that FL118 could inhibit the cell proliferation of both ES-2 and SK-O-V3 cells in a time- and dose-dependent manner.
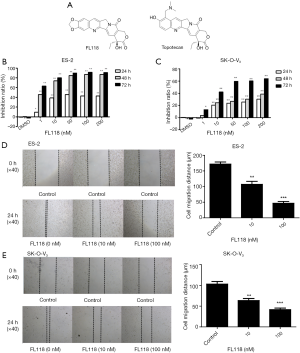
FL118 effectively inhibited the migration of ES-2 and SK-O-V3 cells in a dose-dependent manner
To investigate whether FL118 inhibits the migratory capability of ES-2 and SK-O-V3 cells, different concentrations of FL118 were added to ES-2 and SK-O-V3 cells. After 0 and 24 hours, the cells were photographed. As shown in Figure 1D,E, the cell migration distance of FL118 (100 nM) group was significantly decreased in comparison with FL118 (0, 10 nM) group. These results suggested that FL118 inhibited the migration of ovarian cancer cells in a dose-dependent manner.
FL118 up-regulates the expression of CYGB in ES-2 and SK-O-V3 cells
In order to further elucidate the molecular mechanism of FL118 inhibiting tumor growth, the difference between the control group and FL118 treatment group in protein expression level was analyzed by proteomic analysis technology. Hierarchical clustering was based on expression profiles of significantly differentially expressed genes from control group and drug group in colon cancer (Figure 2). Results showed that CYGB was markedly up-regulated in HCT116 CRC cells with FL118 (100 nM) treatment for 72 hours. Since previous studies have shown that CYGB regulated the cell proliferation and invasion in ovarian cancer (17), the effect of FL118-induced CYGB expression in ES-2 and SK-O-V3 cells was detected by qRT-PCR and immunoblotting assays after FL118 (0, 10, 100 nM) administration for 48 hours. We found that FL118 significantly promoted CYGB expression in mRNA and protein level (Figure 3A,B), which was consistent with the result of proteomic analysis. Moreover, CYGB expression was progressively elevated with an increasing FL118 concentration. Taken together, these data suggested that FL118 could upregulate CYGB expression in ovarian cancer cells in a dose- dependent manner.
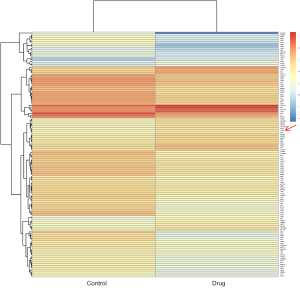
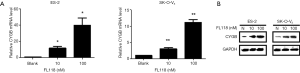
CYGB mediates the antitumor activity of FL118 in ES-2 and SK-O-V3 cells
Subsequently, the role of CYGB in the antitumor activity of FL118 in ES-2 and SK-O-V3 cells was investigated. As shown in Figure 4A,B, CYGB could be inhibited successfully in ES-2 and SK-O-V3 cells by siRNA (si-CYGB). This in turn led to a partial recovery of the cell proliferation and migration of ES-2 and SK-O-V3 cells with FL118 treatment (Figure 4C-E). The above data supported that CYGB was required for FL118 inhibiting tumor growth.
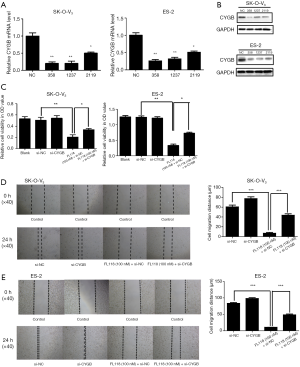
FL118 significantly inhibits PI3K/AKT/mTOR signaling pathway activation, and affects the expression level of vimentin and E-cadherin in ovarian cancer cells
Previous reports have shown that CYGB expression affected the cell migration through the PI3K/AKT/mTOR signaling pathway (21). In this experiment, we investigated if this pathway was activated after treatment with FL118. Western blotting assay was used to detect the expression of phosphorylated and total AKT/mTOR proteins at 48 hours. Results showed that FL118 increased the level of phosphorylated AKT/mTOR proteins (Figure 5), which indicated that FL118 could inhibit PI3K/AKT/mTOR signaling pathway activation in ovarian cancer cells. In addition, cell migration was a well-established phenomenon caused by changes of the expression of protein (E-cadherin/vimentin) in cell, so we investigated if FL118 affected the EMT marker. Results suggested that the protein expression level of E-cadherin/vimentin were increased/decreased respectively (Figure 5), indicating that FL118 could effectively inhibit the migration of ES-2 and SK-O-V3 cells.
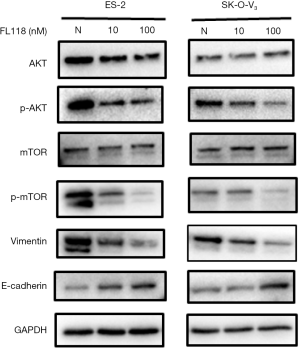
FL118 exhibits better antitumor activity by up-regulating the expression of CYGB compared with topotecan in mice
To further validate the anti-tumor activity of FL118 and its effect on the CYGB expression in vivo, we established a mouse model bearing ES-2 tumor xenograft to compare the inhibitory effect of FL118 with clinically used chemotherapeutic drug topotecan. FL118 was orally administrated once a week for 20 days at two different doses (5 and 10 mg/kg) (18), while topotecan was also administered at its MTD (2 mg/kg per administration) for five times a week for 20 days (20). Both FL118 and topotecan groups decreased the growth of ES-2 tumor (Figure 6A), but FL118 group formed smaller tumors in comparison with topotecan group (Figure 6B,C). More importantly, tumors were abolished completely in 60% of nude mice in 10 mg/kg-dose FL118 group (Figure 6B). Moreover, the average weight of tumors in FL118 group was significantly lower than that in two other groups (Figure 6D). In addition, there was no statistical difference in body weight between the three groups on day 20 (control, topotecan, low-dose FL118) (Figure 6E). However, there was a statistical difference in body weight between control group and high-dose FL118. qRT-PCR and Immunoblotting assay revealed that FL118 up-regulates CYGB expression in ES-2 tumor xenograft (Figure 6F,G). Taken together, these data showed that FL118 could suppress the growth of ES-2 tumors by upregulating CYGB expression and its antitumor activity was superior to topotecan in vivo. Importantly, 10 mg/kg-dose FL118 represented higher toxicity compared with 5 mg/kg-dose FL118.
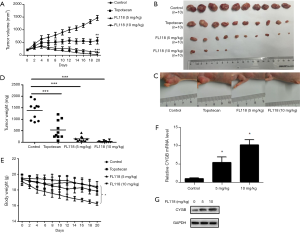
Discussion
Ovarian cancer is one of the deadliest malignant tumors which are highly harmful to women health. Despite the great advances in treatment including using some chemotherapy drugs such as irinotecan and topotecan in recent years, the prognosis of ovarian cancer is still poor due to drug resistance and side effects. Therefore, the development of new anticancer drugs has been always of great interest for researchers. Recently, a novel camptothecin analogue, FL118, has been reported to exert strong antitumor effect and low toxicity on various cancers (10). However, its antitumor effect on ovarian cancer and the mechanism underlying its functions have not been explored yet. In this study, we have found that FL118 could inhibit the cell proliferation and migration of ES-2 and SK-O-V3 cells in a concentration-dependent manner in vitro. Moreover, FL118 exhibits greater antitumor effect in vivo compared to topotecan. It appeared that 5 mg/kg-group had a lower body weight loss in comparison with topotecan-group, although there was no statistical difference between the two groups. In addition, the antitumor effect of 10 mg/kg-group was significant, with almost 60% of the tumor in mice completely removed, but the toxicity was relatively higher than 5 mg/kg-group. We could see that the body weight of 10 mg/kg-group was significantly lower than that of the 5 mg/kg-group or control group, suggesting that the 10 mg/kg-group maybe have certain toxicity to mice.
Despite the similar structures between FL118 and topotecan/irinotecan, the mechanism of action is completely different. It is well-known that topotecan and irinotecan exert their cytotoxicity on cancer cells by targeting topoisomerase 1 (Top1) enzyme and thus inhibiting DNA replication and transcription (22,23), while FL118 was reported not to be a better Top1 inhibitor than irinotecan/topotecan (24). Previous studies showed that inhibition of Top1 by FL118 was mainly involved in cytotoxicity similar to irinotecan and topotecan, rather than its antitumor effect (25). In addition, unlike irinotecan and topotecan, FL118 is not a substrate of the efflux pump proteins ABCG2Jeny (26-29) and P-gp (30,31), suggesting that it may induce lower acquired resistance. Accordingly, FL118 could selectively inhibit other cancer-related proteins besides surviving in a p53-independent manner, including XIAP, clAP2, Mcl-1, Bcl-2 (9). Moreover, studies have revealed that FL118 could function as an MdmX inhibitor and promote p53-dependent apoptosis in colorectal cancer cells (32). Here, we reported CYGB expression was significantly increased after FL118 treatment in ovarian cancer cells, which was consistent with the result of proteomic analysis.
CYGB downregulation and promoter methylation has been reported in many malignancies, such as esophageal, breast and lung cancers (16,33). Previous studies have shown that over-expression of CYGB in breast cancer and non-small lung cancer cells could suppress tumor growth (16). Also, CYGB knockdown has been found to facilitate tumor growth in glioma cells (34) and regulate cell proliferation and invasion in ovarian cancer (17).
Furthermore, in fibroblast cells, CYGB has been reported to inhibit migration through PI3K/AKT/mTOR pathway (21). In this study, we found that CYGB played a role in FL118-mediated ovarian cancer migration and growth inhibition. Results also suggested that FL118 significantly inhibits PI3K/AKT/mTOR signaling pathway activation in ovarian cancer. Our results indicated that CYGB, a tumor suppressor, might participate in FL118’s antitumor activity in vitro and in vivo.
Conclusions
In summary, our work indicated that FL118 effectively inhibits the proliferation and migration of ovarian cancer cells by upregulating CYGB expression in vitro and in vivo. We also found that FL118 significantly inhibits PI3K/AKT/mTOR signaling pathway activation in ovarian cancer. In addition, FL118 exerts greater antitumor effect compared with topotecan in a nude mouse model. These results are of importance for FL118’s further clinical application and elucidating the molecular mechanism underlying its superior antitumor potency.
Acknowledgments
We would like to acknowledge Dr. Fengzhi Li and his team (Roswell Park Cancer Institute-RPCI, Buffalo, NY, USA) for kindly supporting.
Funding: This work was supported by funds from National Natural Sciences Foundation of China (81502065 and 81672926), China Postdoctoral Science Foundation Funded Project (2016T90613, 2015M580574 and 2016M592146), Natural Sciences Foundation of Shandong Province (ZR2014HQ009), Shandong Postdoctoral Innovation Project (201602037), Qingdao Innovation Applied Basic Research Project (16-5-1-56-JCH) and Qingdao Postdoctoral Research Project (2015167 and 2015157).
Footnote
Conflicts of Interest: All authors have completed the ICMJE uniform disclosure form (available at http://dx.doi.org/10.21037/tcr.2017.10.12). The authors have no conflicts of interest to declare.
Ethical Statement: The authors are accountable for all aspects of the work in ensuring that questions related to the accuracy or integrity of any part of the work are appropriately investigated and resolved. The study was conducted in accordance with the Declaration of Helsinki (as revised in 2013). Institutional ethical approval and informed consent were waived. The animal experiment was approved by animal ethical approval (0102470) from Qingdao University.
Open Access Statement: This is an Open Access article distributed in accordance with the Creative Commons Attribution-NonCommercial-NoDerivs 4.0 International License (CC BY-NC-ND 4.0), which permits the non-commercial replication and distribution of the article with the strict proviso that no changes or edits are made and the original work is properly cited (including links to both the formal publication through the relevant DOI and the license). See: https://creativecommons.org/licenses/by-nc-nd/4.0/.
References
- Martino E, Della Volpe S, Terribile E, et al. The long story of camptothecin: From traditional medicine to drugs. Bioorg Med Chem Lett 2017;27:701-7. [Crossref] [PubMed]
- Liew ST, Yang LX. Design, synthesis and development of novel camptothecin drugs. Curr Pharm Des 2008;14:1078-97. [Crossref] [PubMed]
- Li QY, Zu YG, Shi RZ, et al. Review Camptothecin: Current Perspectives. Curr Med Chem 2006;13:2021-39. [Crossref] [PubMed]
- Chen MC, Lee NH, Ho TJ, et al. Resistance to irinotecan (CPT-11) activates epidermal growth factor receptor/nuclear factor kappa B and increases cellular metastasis and autophagy in LoVo colon cancer cells. Cancer Lett 2014;349:51-60. [Crossref] [PubMed]
- Bessho Y, Oguri T, Achiwa H, et al. Role of ABCG2 as a biomarker for predicting resistance to CPT-11/SN-38 in lung cancer. Cancer Sci 2006;97:192-8. [Crossref] [PubMed]
- Leggas M, Adachi M, Scheffer GL, et al. Mrp4 confers resistance to topotecan and protects the brain from chemotherapy. Mol Cell Biol 2004;24:7612-21. [Crossref] [PubMed]
- Cruz-Muñoz W, Di Desidero T, Man S, et al. Analysis of acquired resistance to metronomic oral topotecan chemotherapy plus pazopanib after prolonged preclinical potent responsiveness in advanced ovarian cancer. Angiogenesis 2014;17:661-73. [PubMed]
- Pommier Y. Topoisomerase I inhibitors: camptothecins and beyond. Nat Rev Cancer 2006;6:789-802. [Crossref] [PubMed]
- Ling X, Cao S, Cheng Q, et al. A novel small molecule FL118 that selectively inhibits survivin, Mcl-1, XIAP and cIAP2 in a p53-independent manner, shows superior antitumor activity. PLoS One 2012;7:e45571 [Crossref] [PubMed]
- Zhang DD, Wang J, Yang ZH, et al. A novel camptothecin analogue FL118 reduces cisplatin resistance of non-small cell lung cancer cells. Int J Clin Exp Med 2016;9:13501-13.
- Siegel R, Naishadham D, Jemal A. Cancer statistics, 2013. CA Cancer J Clin 2013;63:11-30. [Crossref] [PubMed]
- Webb PM, Jordan SJ. Epidemiology of epithelial ovarian cancer. Best Pract Res Clin Obstet Gynaecol 2017;41:3-14. [Crossref] [PubMed]
- Presneau N, Dewar K, Forgetta V, et al. Loss of heterozygosity and transcriptome analyses of a 1.2 Mb candidate ovarian cancer tumor suppressor locus region at 17q25.1-q25.2. Mol Carcinog 2005;43:141-54. [Crossref] [PubMed]
- McRonald FE, Liloglou T, Xinarianos G, et al. Down-regulation of the cytoglobin gene, located on 17q25, in tylosis with oesophageal cancer (TOC): evidence for trans-allele repression. Hum Mol Genet 2006;15:1271-7. [Crossref] [PubMed]
- Moodley R, Reddi A, Chetty R, et al. Abnormalities of chromosome 17 in oesophageal cancer. J Clin Pathol 2007;60:990-4. [Crossref] [PubMed]
- Shivapurkar N, Stastny V, Okumura N, et al. Cytoglobin, the newest member of the globin family, functions as a tumor suppressor gene. Cancer Res 2008;68:7448-56. [Crossref] [PubMed]
- Chen H, Zhao X, Meng T. Expression and biological role of cytoglobin in human ovarian cancer. Tumour Biol 2014;35:6933-9. [Crossref] [PubMed]
- Ling X, Liu XJ, Zhong K, et al. FL118, a novel camptothecin analogue, overcomes irinotecan and topotecan resistance in human tumor xenograft models. Am J Transl Res 2015;7:1765-81. [PubMed]
- Westover D, Ling X, Lam H, et al. FL118, a novel camptothecin derivative, is insensitive to ABCG2 expression and shows improved efficacy in comparison with irinotecan in colon and lung cancer models with ABCG2-induced resistance. Mol Cancer 2015;14:92. [Crossref] [PubMed]
- Houghton PJ, Cheshire PJ, Myers L, et al. Evaluation of 9-dimethylaminomethyl-10-hydroxycamptothecin against xenografts derived from adult and childhood solid tumors. Cancer Chemother Pharmacol 1992;31:229-39. [Crossref] [PubMed]
- Demirci S, Doğan A, Apdik H, et al. Cytoglobin inhibits migration through PI3K/AKT/mTOR pathway in fibroblast cells. Mol Cell Biochem 2017; [Epub ahead of print]. [Crossref] [PubMed]
- Hertzberg RP, Caranfa MJ, Hecht SM. On the mechanism of topoisomerase I inhibition by camptothecin: evidence for binding to an enzyme-DNA complex. Biochemistry 1989;28:4629-38. [Crossref] [PubMed]
- Li TK, Liu LF. Tumor cell death induced by topoisomerase-targeting drugs. Annu Rev Pharmacol Toxicol 2001;41:53-77. [Crossref] [PubMed]
- Zhao J, Ling X, Cao S, et al. Antitumor activity of FL118, a survivin, Mcl-1, XIAP, and cIAP2 selective inhibitor, is highly dependent on its primary structure and steric configuration. Mol Pharm 2014;11:457-67. [Crossref] [PubMed]
- Li F, Ling X, Harris DL, et al. Topoisomerase I (Top1): a major target of FL118 for its antitumor efficacy or mainly involved in its side effects of hematopoietic toxicity? Am J Cancer Res 2017;7:370-82. [PubMed]
- Filipski E, Berland E, Ozturk N, et al. Optimization of irinotecan chronotherapy with P-glycoprotein inhibition. Toxicol Appl Pharmacol 2014;274:471-9. [Crossref] [PubMed]
- Kruijtzer CMF, Beijnen JH, Rosing H, et al. Increased oral bioavailability of topotecan in combination with the breast cancer resistance protein and P-glycoprotein inhibitor GF120918. J Clin Oncol 2002;20:2943-50. [Crossref] [PubMed]
- Ling X, Xu C, Fan C, et al. FL118 induces p53-dependent senescence in colorectal cancer cells by promoting degradation of MdmX. Cancer Res 2014;74:7487-97. [Crossref] [PubMed]
- Xinarianos G, McRonald FE, Risk JM, et al. Frequent genetic and epigenetic abnormalities contribute to the deregulation of cytoglobin in non-small cell lung cancer. Hum Mol Genet 2006;15:2038-44. [Crossref] [PubMed]
- Fang J, Ma I, Allalunis-Turner J. Knockdown of cytoglobin expression sensitizes human glioma cells to radiation and oxidative stress. Radiat Res 2011;176:198-207. [Crossref] [PubMed]
- Shishido Y, Ueno S, Yamazaki R, et al. ABCG2 Inhibitor YHO-13351 Sensitizes Cancer Stem/Initiating-like Side Population Cells to Irinotecan. Anticancer Res 2013;33:1379-86. [PubMed]
- Su Y, Lee SH, Sinko PJ. Inhibition of efflux transporter ABCG2/BCRP does not restore mitoxantrone sensitivity in irinotecan-selected human leukemia CPT-K5 cells: Evidence for multifactorial multidrug resistance. Eur J Pharm Sci 2006;29:102-10. [Crossref] [PubMed]
- Yoshikawa M, Ikegami Y, Sano K, et al. Transport of SN-38 by the wild type of human ABC transporter ABCG2 and its inhibition by quercetin, a natural flavonoid. J Exp Ther Oncol 2004;4:25-35. [PubMed]
- Houghton PJ, Germain GS, Harwood FC, et al. Imatinib mesylate is a potent inhibitor of the ABCG2 (BCRP) transporter and reverses resistance to topotecan and SN-38 in vitro. Cancer Research 2004;64:2333-7. [Crossref] [PubMed]