Elevated expression of mitochondrial transcription elongation factor (TEFM) predicts poor prognosis in low grade glioma—an analysis of the Cancer Genome Atlas (TCGA) dataset
Introduction
Low grade glioma (LGG) is a group of heterogeneous neuroepithelial tumors arising from supporting glial cells of the central nervous system (1). The World Health Organization (WHO) has traditionally classified gliomas in four grades, according to histopathologic features like atypia, anaplasia, mitotic activity, microvascular proliferation and the presence of necrosis (2,3). These tumors seem to grow slow but continuously over time, usually along the white matter fibers of the hemispheres (4,5). Once malignant differentiation occurs, they behave as WHO grade III or IV tumors and a rapid neurological deterioration is expected before death (5). Currently, the mean survival of LGGs is generally less than 10 years from diagnosis (5). Therefore, it is of critical significance to identify and characterized novel markers in order to investigate new therapeutic approaches and improve patients’ clinical outcome.
Mitochondrial function is critical for the survival of eukaryotes. The main functions of mitochondria are to generate ATP, conduct biosynthesis, and cause cell death (including apoptosis and programmed cell necrosis) (6-8). In addition, mitochondria are also involved in important physiological processes such as the tricarboxylic acid cycle, oxidation of fatty acids and amino acids, and steady state regulation of calcium ions (9). Mitochondrial dysfunctions are involved in numerous human diseases, such as neurodegenerative diseases, heart diseases, diabetes, and cancer (10-12). An essential process for a normal mitochondrial function is mitochondrial gene expression which is tightly regulated in response to various physiological changes. The accurate control of mitochondrial gene expression is essential in order to provide the appropriate oxidative phosphorylation (OXPHOS) capacity for diverse metabolic demands. Human mitochondrial transcription elongation factor (TEFM) is also named C17orf42, which is an important protein factor involved in the regulation of mitochondrial gene transcription (13). TEFM gene is located within the region of 17q11.2 and contains 4 exons. Its mRNA transcript (NM_024683.3) is 1,357 bp in length and encodes a protein consisting of 360 amino acid residues, with 1–35 amino acid residues at the N-terminal domain as its mitochondrial targeting sequences (MTS) (14). Previous studies have shown that TEFM is a key molecule for human mitochondrial DNA (mtDNA) replication-transcription switch (15). TEFM protein can specifically bind to mitochondrial RNA polymerase, and can significantly enhance the transcription extension activity of mtDNA both in vivo and in vitro (16,17). To the best of our knowledge, the expression of TEFM gene in LGG and its relationship with the development and prognosis of LGG patients have not been reported.
This study was based on the Cancer Genome Atlas (TCGA) dataset to explore the relationship between the expression levels of TEFM gene and the clinicopathological characteristics of LGG patients and its prognostic significance. The role and mechanism of occurrence and development and prognosis provide theoretical basis, and also provide new ideas for gene targeted therapy of LGG.
Methods
Data collection from the TCGA database
The RNA-Seq expression (lgg_tcga_rna_seq_v2_mrna, combining level 3 data from Illumina GA and HiSeq platform) and clinical data for LGG patients were downloaded from TCGA data portal (http://cancergenome.nih.gov/) by using the Cgdsr package of R3.6.1 software. The methods of biospecimen procurement, RNA isolation, and RNA sequencing were previously described by TCGA Research Network (18). All 530 LGG patients in the TCGA database were given a follow-up for 0 to 182 months. For the analysis of survival and follow-up, the data of surgery was used as the beginning of the follow-up period. The endpoint of the patient cohort analysis was the time to death or the date of the last visit (if no death occurred). All patients who died of diseases or accidents other than LGG were excluded from cohort.
Gene Expression Profiling Interactive Analysis (GEPIA) analysis of the difference of TEFM expression between LGG and non-tumor brain tissue in TCGA dataset
GEPIA is an online application tool that can be used to analyze the differential expression of genes in cancer and normal tissues. The TCGA and Genotype-Tissue expression (GTEx) datasets dataset includes 518 LGG tissue samples and 207 non-tumor brain tissue samples. GEPIA was used to analyze the expression difference of TEFM gene in LGG and non-tumor brain tissue.
Dataset screening and clinical pathological parameters of LGG
The data from a total of 530 LGG tissues were downloaded by using R3.6.1 software. The clinical data of the LGG patients in the TCGA dataset were filtered to keep cases with clinical parameters and survival data. After the dataset was filtered, 463 cases with complete clinical parameters and survival data were incorporated. According to the second-generation mRNA-sequencing data, the expression of TEFM mRNA in LGG tissues ranged from 60.1915 to 290.2066, with a median of 119.048. If the expression of TEFM mRNA in an LGG tissue sample was greater than the median, it was defined as a high expression of TEFM; otherwise, it was considered a low expression of TEFM. Accordingly, TEFM mRNA was highly expressed in 231 cases and lowly expressed in 232 cases. The detailed information on the clinical features were quantitatively assigned in Table 1.
Table 1
Influencing factors | Assignment explanation |
---|---|
Age | ≤50 years old =1, >50 years old =2 |
Sex | Male =1, female =2 |
WHO grade | G2 =1, G3 =2 |
Pathological type | Anaplastic astrocytoma =1, anaplastic oligoastrocytoma =2 astrocytoma =3, oligoastrocytoma =4, oligodendroglioma =5 |
Headache history | Without =0, with =1 |
Histological diagnosis | Astrocytoma =1, oligoastrocytoma =2, oligodendroglioma =3 |
Laterality | Left =1, middle =2, right =3 |
Supratentorial location | Cerebral cortex =1, white matter =2, other =3 |
Tumor location | Frontal lobe =1, occipital lobe =2, parietal leaf =3, temporal lobe =4, other =5 |
TEFM expression | Low expression of TEFM =1, high expression of TEFM =2 |
Survival time | Actual survival time (months) |
Patient outcome | Alive =0, death =1 |
LGG, low grade glioma; WHO, World Health Organization; TEFM, mitochondrial transcription elongation factor.
Correlation analysis of gene expression in LGG
GEPIA analysis were used to analyze the correlation between mRNA expression levels of TEFM gene and mRNA expression levels of other related mitochondrial regulatory genes in LGG. In this study, the selection of mitochondrial regulatory genes included: mitochondrial transcription factor A (TFAM); mitochondrial transcription factor B1 (TFB1M); mitochondrial transcription factor B2 (TFB2M); mitochondrial transcription termination factor 1 (MTERF1); mitochondrial transcription termination factor 2 (MTERF2); mitochondrial transcription termination factor 3 (MTERF3); mitochondrial transcription termination factor 4 (MTERF4); nuclear respiratory factor 1 (NRF1) and mitochondrial RNA polymerase (POLRMT) were analyzed, respectively.
Gene set enrichment analysis (GSEA)
GSEA was performed using the Molecular Signatures Database (MSigDB) software, which was obtained from the Broad Institute (http://www.broad.mit.edu/gsea), as previously described (19,20). The goal of GSEA in our research was to identify the distribution of biological functions within the differentially expressed genes (DEGs). Enrichment map was used for visualization of the GSEA results. False discovery rate (FDR) value and normalized enrichment score (NES) were used to sort the pathways enriched in each phenotype after gene set permutations were performed 1,000 times for each analysis.
Approval was waived by the local ethics committee, as TCGA database, GEPIA online tool and GSEA software are publicly available and de-identified.
Statistical analysis
The patients’ clinical data, such as the patients’ age, sex, WHO grade, pathological type, headache history, histological diagnosis, laterality, tumor location, TEFM mRNA expression and other quantitative indicators analysis were quantitatively assigned after the establishment of the patient clinical database. Statistical analysis was carried out by SPSS 22.0 software (SPSS, Inc., Chicago, IL, USA) and GraphPad Prism 7.01 (GraphPad Software, La Jolla, CA, USA). The independent Student’s t-test was used to analyze the results and data expressed as the mean ± standard deviation (SD). The categorical data were summarized with frequencies and percentage. Shapiro-Wilk method was used to detect the normal distribution of TEFM mRNA expression in LGG tissues. The results showed that the TEFM mRNA expression level did not conform to the normal distribution, so it was tested by using a Mann-Whitney U non-parametric test. Correlation analysis of TEFM expression and clinical pathological parameters by using χ2 test and Fisher’s exact probability method. Using the Survplot package of R3.6.1 software, Kaplan-Meier method was used to plotted survival curve and log-rank test was performed. In consideration of other confounding factors and the impact of a suppressor effect, variables with a significant value of P<0.1 were subjected to a multivariate Cox proportional hazard analysis and further screened by forward selection method to evaluate their independent effect. The Cox proportional hazard regression models were used for multivariate analysis, and the relative risks of dying were expressed as adjusted hazard ratios (HRs) and corresponding 95% confidence intervals (CIs). Pearson’s analysis was used to analyze the correlation of gene expression. All P values resulted from two-sided statistical testing. And a P<0.05 was considered to reveal a statistically significant difference, and P<0.01 referred to highly significant difference.
Results
TEFM mRNA was upregulated in LGG tissues
GEPIA is an online tool based on TCGA and GTEx data. The LGG dataset of TCGA was visually analyzed by GEPIA. The results showed that the expression of TEFM mRNA in human LGG tissues (n=518) were significantly higher than that in non-tumor brain tissues (n=207). There was significant difference between LGG tissues and non-tumor brain tissues (P<0.05; Figure 1).
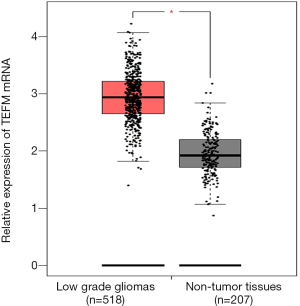
Correlation of TEFM mRNA expression and clinicopathological characteristics in LGG patients
In the LGG dataset of TCGA, a total of 463 samples with complete clinicopathological parameters were available for analysis. The prognostic influencing factors of LGG patients were summarized in Table 1. Median age of this patient cohort was 41.0 years (range, 14.0–87.0 years). According to statistical analysis, we found that TEFM mRNA expression were significantly related with the age (P=0.024), WHO grade (P<0.001), pathological types (P=0.004), headache history (P=0.022), and supratentorial location (P=0.009) of LGG patients. There was no significant correlation with sex, histological diagnosis, laterality and tumor location (all P>0.05; Table 2). TEFM was upregulated in LGG tissues with older age, higher grade patients (Figure 2A,B). Moreover, the expression of TEFM mRNA was significantly upregulated in oligoastrocytoma and oligodendroglioma, compared with anaplastic astrocytoma (Figure 2C). The expression of TEFM mRNA was significantly upregulated in white matter and other location, compared with cerebral cortex (Figure 2D).
Table 2
Clinical indicators | N | TEFM expression, n (%) | χ2 | P value | |
---|---|---|---|---|---|
Low | High | ||||
Age (years) | 5.092 | 0.024 | |||
≤50 | 323 | 173 (53.56) | 150 (46.44) | ||
>50 | 140 | 59 (42.14) | 81 (57.86) | ||
Sex | 0.372 | 0.541 | |||
Male | 254 | 124 (48.82) | 130 (51.18) | ||
Female | 209 | 108 (51.67) | 101 (48.33) | ||
WHO grade | 13.523 | <0.001 | |||
G2 | 222 | 131 (59.01) | 91 (40.99) | ||
G3 | 241 | 101 (41.91) | 140 (58.09) | ||
Pathological type | 15.341 | 0.004 | |||
Anaplastic astrocytoma | 116 | 48 (41.38) | 68 (58.62) | ||
Anaplastic oligoastrocytoma | 71 | 27 (38.03) | 44 (61.97) | ||
Astrocytoma | 57 | 36 (63.16) | 21 (36.84) | ||
Oligoastrocytoma | 115 | 68 (59.13) | 47 (40.87) | ||
Oligodendroglioma | 104 | 53 (50.96) | 51 (49.04) | ||
Headache history | 5.220 | 0.022 | |||
With | 168 | 96 (57.14) | 72 (42.86) | ||
Without | 295 | 136 (46.10) | 159 (53.90) | ||
Histological diagnosis | 5.260 | 0.072 | |||
Astrocytoma | 173 | 84 (48.55) | 89 (51.45) | ||
Oligoastrocytoma | 115 | 68 (59.13) | 47 (40.87) | ||
Oligodendroglioma | 175 | 80 (45.71) | 95 (54.29) | ||
Laterality | 0.282 | 0.871 | |||
Left | 233 | 118 (50.64) | 115 (49.36) | ||
Middle | 5 | 3 (60.00) | 2 (40.00) | ||
Right | 225 | 111 (49.33) | 114 (50.67) | ||
Supratentorial location | 9.451 | 0.009 | |||
Cerebral cortex | 138 | 60 (43.48) | 78 (56.52) | ||
White matter | 107 | 67 (62.62) | 40 (37.38) | ||
Other | 218 | 105 (48.17) | 113 (51.83) | ||
Tumor location | 1.910 | 0.753 | |||
Frontal lobe | 273 | 134 (49.08) | 139 (50.92) | ||
Occipital lobe | 5 | 2 (40.00) | 3 (60.00) | ||
Parietal leaf | 41 | 23 (56.10) | 18 (43.90) | ||
Temporal lobe | 133 | 69 (51.88) | 64 (48.12) | ||
Other | 11 | 4 (36.36) | 7 (63.64) |
TEFM, mitochondrial transcription elongation factor; LGG, low grade glioma; WHO, World Health Organization.

High expression of TEFM mRNA was correlated with poor survival in LGG patients
Disease-free survival (DFS), as well as the overall survival (OS), is of importance to LGG patients. To assess the prognostic value of TEFM expression in LGG, we next analyzed its correlation with OS and DFS. Differences in the survival time between high expression and low expression of TEFM in TCGA dataset were compared by Kaplan-Meier method, with p-values calculated via log-rank test, using the Survplot package of R3.6.1 software. The results showed that mRNA expression of TEFM was significantly correlated with OS (HR =1.45; 95% CI: 1.01–2.08; log-rank P=0.047; Figure 3A), but not significantly correlated with DFS (HR =1.06; 95% CI: 0.78–1.45; log-rank P=0.692) in LGG patients (Figure 3B). Higher levels of TEFM expression were associated with a reduced OS, with median survival of 67.41 months, compared to the period of 95.50 months for the low TEFM expression group. However, the median DFS time of the TEFM high expression group (41.95 months) was not significantly lower than that of low expression group (42.90 months).
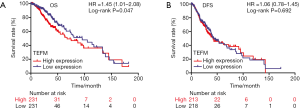
Multivariate Cox regression analysis the prognosis of LGG patients
The factors affecting the prognosis of LGG patients were analyzed by univariate analysis and multivariate Cox analysis. The results of univariate analysis showed that the age, WHO grade, pathological type, supratentorial location and expression of TEFM significantly affected the prognosis of LGG patients (all P<0.05). However, factors such as sex, headache history and laterality did not affect the prognosis of LGG patients (all P>0.05). Factors with P<0.1 in the above analysis were included in a Cox regression model for a multivariate analysis. The corresponding results suggested that age, WHO grade, pathological type and supratentorial location were independent factors affecting prognosis of LGG patients (all P<0.05; Table 3).
Table 3
Clinicopathological characteristics | Univariate analysis | Multivariate analysis | |||||
---|---|---|---|---|---|---|---|
HR | 95% CI | P | HR | 95% CI | P | ||
Age (≤50 vs. >50 years) | 3.31 | 2.26–4.85 | <0.001 | 3.40 | 2.25–5.13 | <0.001 | |
Sex (male vs. female) | 1.09 | 0.75–1.57 | 0.645 | 1.00 | 0.68–1.45 | 0.978 | |
WHO grade (G3 vs. G2) | 3.62 | 2.38–5.50 | <0.001 | 2.95 | 1.45–5.99 | 0.003 | |
Pathological type (vs. anaplastic astrocytoma) | |||||||
Anaplastic oligoastrocytoma | 0.56 | 0.33–0.96 | 0.035 | 0.40 | 0.23–0.90 | 0.001 | |
Astrocytoma | 0.23 | 0.11–0.47 | <0.001 | 0.73 | 0.27–2.03 | 0.553 | |
Oligoastrocytoma | 0.37 | 0.22–0.62 | <0.001 | 0.63 | 0.35–1.13 | 0.123 | |
Oligodendroglioma | 0.23 | 0.13–0.41 | <0.001 | 0.51 | 0.23–1.16 | 0.107 | |
Headache history (with vs. without) | 0.85 | 0.58–1.25 | 0.413 | 0.94 | 0.63–1.42 | 0.771 | |
Laterality (vs. left) | |||||||
Middle | 0.68 | 0.16–2.92 | 0.602 | 0.82 | 0.17–3.95 | 0.800 | |
Right | 0.72 | 0.50–1.05 | 0.084 | 0.70 | 0.47–1.04 | 0.076 | |
Supratentorial location (vs. cerebral cortex) | |||||||
White matter | 1.65 | 0.94–2.89 | 0.080 | 1.96 | 1.08–3.53 | 0.026 | |
Other | 1.93 | 1.22–3.06 | 0.005 | 1.92 | 1.20–3.08 | 0.007 | |
TEFM expression (high vs. low) | 1.45 | 1.01–2.09 | 0.045 | 1.32 | 0.90–1.94 | 0.161 |
LGG, low grade glioma; WHO, World Health Organization; HR, hazard ratio; CI, confidence interval; TEFM, mitochondrial transcription elongation factor.
Correlation between the expression of the TEFM gene and other mitochondrial transcription regulatory genes in LGG
The results of Pearson’s correlation analysis showed that the expression levels of the TEFM gene in LGG samples were positively correlated with the expression levels of TFAM (P=0, R=0.59), TFB1M (P=0, R=0.51), TFB2M (P=0, R=0.68), MTERF1 (P=0, R=0.70), MTERF2 (P=0, R=0.36), MTERF3 (P=0, R=0.51), MTERF4 (P=0, R=0.51) and NRF1 (P=0, R=0.48) genes, but negatively correlated with the expression levels of the POLRMT gene (P=5.6e−05, R=−0.18; Figure 4).
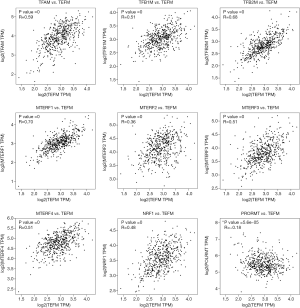
Identification of TEFM-associated biological pathways by GSEA
To identify TEFM-associated biological signaling pathways on an unbiased basis, we performed GSEA using high throughput RNA-sequencing data of the TCGA cohort. The expression level of TEFM was used as the phenotype label. Among all the predefined Kyoto Encyclopedia of Genes and Genomes (KEGG) pathways gene sets, cell cycle, RNA degradation, spliceosome and ubiquitin mediated proteolysis were found to be significantly associated with TEFM expression in the TCGA cohort (Figure 5), suggesting that TEFM may be involved in LGG development and progression through the above cancer-associated signaling pathways.
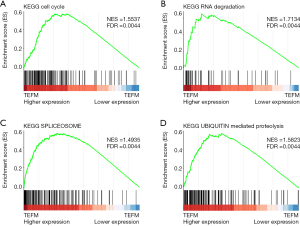
Discussion
LGG is typically slow-growing gliomas having moderately increased cellularity generally without mitosis, necrosis, and microvascular proliferation, and there is no clear demarcation between glioma and non-tumor brain tissue, which significantly increases the difficulty of surgical resection (1-5). This is the main reason for the low survival rate and poor prognosis of glioma patients (21). In addition, glioma tissue has the characteristics of continuous and infinite replication, rapid angiogenesis, and immune escape, so it has a strong ability to invade and metastasize (22). Glioma is a highly heterogeneous tumor, and it is difficult to accurately locate it with chemoradiotherapy drugs, which results in glioma cells being highly resistant to chemoradiotherapy (23). Previous studies have found that the occurrence of malignant tumors is not only related to genetic material in the nucleus, but also to mtDNA outside the nucleus (24). Furthermore, the products of nuclear genes involved in mitochondrial replication and transcription molecules act as communication integrators between mitochondria and nucleus, playing an essential role in both homeostasis and tumorigenesis (25). Since the generalized acceptance of the Warburg effect as the mechanism underlying decreased OXPHOS and increased aerobic glycolytic energy production in cancer cells, a strong correlation has been found between mitochondrial dysfunction, represented by mitochondrial protein and DNA mutations and changes mtDNA content, and human cancers (26,27). The occurrence of malignant tumors is not only related to structural changes of mtDNA, but also closely related to the copy numbers and the expression levels of mtDNA. Increased mitochondrial RNA in pre-malignant colon lesion was first reported in 1989 (28). Thereafter a significant increase in elevated mtDNA copy number was demonstrated in endometrial adenocarcinoma (29), laryngeal squamous cell carcinoma (30), and thyroid tumors (31) compared to respective normal tissue. In contrast, mtDNA copy number and mitochondrial respiratory protein reduction were observed in hepatocellular carcinoma (32), ovarian carcinoma (33), breast carcinoma (34), and diffusely infiltrating astrocytomas (35). Furthermore, previous studies have suggested that mutations in mitochondrial genes and changes in mitochondrial dynamics are closely related to the occurrence and development of gliomas (36,37). As we known, TFAM is essential for the replication, transcription, and maintenance of mtDNA and, therefore, for mitochondrial homeostasis (38). In human glioma, evidence indicates that mRNA and protein levels of TFAM are upregulated, compared to non-tumor brain tissue. Moreover, the protein levels of TFAM positively correlated with the malignancy of gliomas (39). However, the relationship between changes in mitochondrial gene transcription levels and the occurrence and development of gliomas remains to be further clarified. As a necessary factor for regulating transcription elongation of mitochondrial genes, expression of TEFM in LGG, its role in the occurrence and development of LGG, and its relationship with the prognosis of LGG have not been reported.
In this study, we firstly discovered that the expression level of TEFM mRNA was significantly increased in LGG tissues compared to those in non-tumor brain tissues, and the difference was statistically significant (P<0.05). Next, we used R3.6.1 software to download RNA SeqV2 data and corresponding clinicopathological data of 530 LGG tissues from the TCGA database. After processing, we obtained TEFM mRNA expression and clinical pathological data of 463 LGG patients. Statistical analysis showed that the expression of TEFM mRNA was significantly correlated with the patient’s age, WHO grade, pathological type, headache history, and supratentorial location in LGG patients. However, high TEFM levels were not associated with the patient’s sex, histological diagnosis, laterality, and tumor location. In addition, high expression of TEFM was dramatically associated with the old age, high WHO grade, advanced tumor status and short OS time. The above results suggest that the TEFM gene plays an important role in tumor progression in LGG as an oncogene. TEFM may be involved in the aggressive tumor progression of LGG patients, and it is related to the malignancy of the tumor. More importantly, TEFM expression was identified as an unfavorable prognostic factor of OS in LGG patients. In other words, the cumulative survival of LGG patients with high expression of TEFM was significantly shorter compared to those with low expression of TEFM. However, there was no significant difference between the two groups with DFS in LGG patients. The pathological factors that may affect the prognosis of LGG patients were further included in the Cox proportional hazard regression model, and univariate and multivariate analysis were performed. Univariate analysis showed that age, WHO grade, pathological type, supratentorial location, and TEFM mRNA expression of LGG patients may affect the prognosis of patients, while patients’ sex, headache history and laterality did not affect the prognosis. The results of multivariate Cox analysis suggested that age, WHO grade, pathological type, and supratentorial location were independent factors affecting the prognosis of LGG patients. Subsequently, we used GEPIA to analyze the correlation between the expression levels of TEFM gene and other mitochondrial transcription regulatory genes in LGG. We found that there was a significantly positive correlation between the expression levels of TEFM gene and TFAM, TFB1M, TFB2M, MTERF1–F4, NRF1 genes (all P<0.01, R>0). There was a significantly negative correlation between the expression level of TEFM gene and POLRMT gene (P=5.6e−05, R=−0.18) in LGG. The above results suggested that the TEFM gene may be co-expressed with other mitochondrial transcription regulatory genes and participate in the regulation of mitochondrial copy number and transcriptional level in LGG, which in turn affects intracellular energy metabolism. It is worth noting that the expression of TEFM gene is negatively correlated with the expression of POLRMT gene in LGG. It is suggested that the synergistic effect of these two genes in malignant tumor cells may be different from that in normal tissues and cells, which needs to be further study. Finally, GSEA results showed that cell cycle, RNA degradation, spliceosome and ubiquitin mediated proteolysis were found to be significantly associated with TEFM expression in the TCGA cohort, suggesting that TEFM may be involved in LGG occurrence and development through the above cancer-associated signaling pathways.
Several drawbacks exist in this study. Firstly, our analysis utilized RNA-seq expression from LGG samples as reported by the TCGA, and protein data were not available to confirm these expression levels. Nonetheless, the uniform accuracy and precision of an integrated analysis as performed by TCGA Research Network, as well as its comprehensive clinical data, add strength to this study. In the follow-up study, we will use Western blot and immunohistochemistry to further analyze the expression of TEFM protein in LGG tissues. Secondly, data regarding recurrence sites and treatment modality are not available and limit the clinical outcome analysis of this study. Lastly, we were unable to analyze the molecular typing of LGG, and the correlation of TEFM expression with clinical biomarkers of LGG, as these expression data of biomarkers were not recorded in this TCGA dataset, thus limiting our conclusion of the role of TEFM in LGG.
In summary, our data have provided evidence that TEFM is upregulated in human LGG. We also document for the first time that high expression of TEFM associates with the aggressive progression and poor prognosis in LGG patients, highlighting its potential as a prognostic marker and a novel molecular target for LGG treatment. Therefore, basic experiments in vitro and in vivo and large samples of patients with long-term follow-up outcomes are needed to confirm the effects of TEFM in LGG in the future.
Acknowledgments
Funding: This study was financially supported by
Footnote
Conflicts of Interest: All authors have completed the ICMJE uniform disclosure form (available at http://dx.doi.org/10.21037/tcr.2020.04.16). The authors have no conflicts of interest to declare.
Ethical Statement: The authors are accountable for all aspects of the work in ensuring that questions related to the accuracy or integrity of any part of the work are appropriately investigated and resolved. This study was approved by the Ethical Committee of College of Basic Medical Sciences, Dali University (ID: 2017-1201).
Open Access Statement: This is an Open Access article distributed in accordance with the Creative Commons Attribution-NonCommercial-NoDerivs 4.0 International License (CC BY-NC-ND 4.0), which permits the non-commercial replication and distribution of the article with the strict proviso that no changes or edits are made and the original work is properly cited (including links to both the formal publication through the relevant DOI and the license). See: https://creativecommons.org/licenses/by-nc-nd/4.0/.
References
- Kleihues P, Louis DN, Scheithauer BW, et al. The WHO classification of tumors of the nervous system. J Neuropathol Exp Neurol 2002;61:215-25. [Crossref] [PubMed]
- Louis DN, Ohgaki H, Wiestler OD, et al. The 2007 WHO classification of tumours of the central nervous system. Acta Neuropathol 2007;114:97-109. [Crossref] [PubMed]
- Komori T. The 2016 WHO classification of tumours of the central nervous system: the major points of revision. Neurol Med Chir (Tokyo) 2017;57:301-11. [Crossref] [PubMed]
- Duffau H, Taillandier L. New concepts in the management of diffuse low-grade glioma: proposal of a multistage and individualized therapeutic approach. Neuro Oncol 2015;17:332-42. [PubMed]
- Wessels PH, Weber WE, Raven G, et al. Supratentorial grade II astrocytoma: biological features and clinical course. Lancet Neurol 2003;2:395-403. [Crossref] [PubMed]
- Wagner BK, Kitami T, Gilbert TJ, et al. Large-scale chemical dissection of mitochondrial function. Nat Biotechnol 2008;26:343-51. [Crossref] [PubMed]
- Soriano ME, Scorrano L. Traveling Bax and Forth from Mitochondria to Control Apoptosis. Cell 2011;145:15-7. [Crossref] [PubMed]
- Hengartner MO. The biochemistry of apoptosis. Nature 2000;407:770-6. [Crossref] [PubMed]
- Detmer SA, Chan DC. Functions and dysfunctions of mitochondrial dynamics. Nat Rev Mol Cell Biol 2007;8:870-9. [Crossref] [PubMed]
- Heller A, Brockhoff G, Goepferich A. Targeting drugs to mitochondria. Eur J Pharm Biopharm 2012;82:1-18. [Crossref] [PubMed]
- Abramov AY, Smulderssrinivasan TK, Kirby DM, et al. Mechanism of neurodegeneration of neurons with mitochondrial DNA mutations. Brain 2010;133:797-807. [Crossref] [PubMed]
- Weinberg SE, Chandel NS. Targeting mitochondria metabolism for cancer therapy. Nat Chem Biol 2015;11:9-15. [Crossref] [PubMed]
- Minczuk M, He J, Duch A M, et al. TEFM (c17ofr42) is necessary for transcription of human mtDNA. Nucleic Acid Res 2011;39:4284-99. [Crossref] [PubMed]
- Hillen HS, Parshin AV, Agaronyan K, et al. Mechanism of transcription anti-termination in human mitochondria. Cell 2017;171:1082-1093.e13. [Crossref] [PubMed]
- Agaronyan K, Morozov YI, Anikin M, et al. Replication-transcription switch in human mitochondria. Science 2015;347:548-51. [Crossref] [PubMed]
- Posse V, Shahzad S, Falkenberg M, et al. TEFM is a potent stimulator of mitochondrial transcription elongation factor in vitro. Nucleic Acid Res 2015;43:2615-24. [Crossref] [PubMed]
- Jiang S, Koolmeister C, Misic J, et al. TEFM regulates both transcription elongation and RNA processing in mitochondria. EMBO Rep 2019;20:e48101. [Crossref] [PubMed]
- Patel SH, Poisson LM, Brat DJ, et al. T2-FLAIR mismatch, an imaging biomarker for IDH and 1p/19q status in lower-grade gliomas: a TCGA/TCIA project. Clin Cancer Res 2017;23:6078-85. [Crossref] [PubMed]
- Kapoor A, Yao W, Ying H, et al. Yap1 activation enables bypass of oncogenic Kras addiction in pancreatic cancer. Cell 2014;158:185-97. [Crossref] [PubMed]
- Yuan JH, Yang F, Wang F, et al. A long noncoding RNA activated by TGF-beta promotes the invasion-metastasis cascade in hepatocellular carcinoma. Cancer Cell 2014;25:666-81. [Crossref] [PubMed]
- Bulakbaşı N, Paksoy Y. Advanced imaging in adult diffusely infiltrating low-grade gliomas. Insights Imaging 2019;10:122. [Crossref] [PubMed]
- Hanahan D, Weinberg RA. Hallmarks of cancer: the next generation. Cell 2011;144:646-74. [Crossref] [PubMed]
- Hadjipanayis CG, Van Meir EG. Tumor initiating cells in malignant gliomas: biology and implications for therapy. J Mol Med (Berl) 2009;87:363-74. [Crossref] [PubMed]
- Reznik E, Miller ML, Şenbabaoğlu Y, et al. Mitochondrial DNA copy number variation across human cancers. Elife 2016;5:e10769. [Crossref] [PubMed]
- Zi J, Wang W, Sun M, et al. A high expression of MTERF3 correlates with tumor progression and predicts poor outcomes in patients with brain glioma. Int J Clin Exp Pathol 2019;12:1909-20. [PubMed]
- Cui H, Huang P, Wang ZJ, et al. Association of decreased mitochondrial DNA content with the progression of colorectal cancer. BMC Cancer 2013;13:110. [Crossref] [PubMed]
- Luna B, Bhatia S, Yoo C, et al. Proteomic and mitochondrial genomic analyses of pediatric brain tumors. Mol Neurobiol 2015;52:1341-63. [Crossref] [PubMed]
- Yamamoto J, Matsumoto K, Furuya T. Lymphangioma of the ascending colon. J Clin Gastroenterol 1989;11:598-9. [Crossref] [PubMed]
- Wang Y, Liu VW, Xue WC, et al. The increase of mitochondrial DNA content in endometrial adenocarcinoma cells: a quantitative study using laser-captured microdissected tissues. Gynecol Oncol 2005;98:104-10. [Crossref] [PubMed]
- Guo W, Yang D, Xu H, et al. Mutations in the D-loop region and increased copy number of mitochondrial DNA in human laryngeal squamous cell carcinoma. Mol Biol Rep 2013;40:13-20. [Crossref] [PubMed]
- Mambo E, Chatterjee A, Xing M, et al. Tumor-specific changes in mtDNA content in human cancer. Int J Cancer 2005;116:920-4. [Crossref] [PubMed]
- Yin PH, Lee HC, Chau GY, et al. Alteration of the copy number and deletion of mitochondrial DNA in human hepatocellular carcinoma. Br J Cancer 2004;90:2390-6. [Crossref] [PubMed]
- Wang Y, Liu VW, Xue WC, et al. Association of decreased mitochondrial DNA content with ovarian cancer progression. Br J Cancer 2006;95:1087-91. [Crossref] [PubMed]
- Yu M, Zhou Y, Shi Y, et al. Reduced mitochondrial DNA copy number is correlated with tumor progression and prognosis in chinese breast cancer patients. IUBMB Life 2007;59:450-7. [Crossref] [PubMed]
- Correia RL, Oba-Shinjo SM, Uno M, et al. Mitochondrial DNA depletion and its correlation with TFAM, TFB1M, TFB2M and POLG in human diffusely infiltrating astrocytomas. Mitochondrion 2011;11:48-53. [Crossref] [PubMed]
- Chen X, Li S, Ke Y, et al. KLF16 suppresses human glioma cell proliferation and tumourigenicity by targeting TFAM. Artif Cells Nanomed Biotechnol 2018;46:608-15. [Crossref] [PubMed]
- DeHaan C, Habibi-Nazhad B, Yan E, et al. Mutation in mitochondrial complex I ND6 subunit is associated with defective response to hypoxia in human glioma cells. Mol Cancer 2004;3:19-33. [Crossref] [PubMed]
- Xie Q, Wu Q, Horbinski CM, et al. Mitochondrial control by DRP1 in brain tumor initiating cells. Nat Neurosci 2015;18:501-10. [Crossref] [PubMed]
- Franco DG, Moretti IF, Marie SKN. Mitochondria transcription factor A: a putative target for the effect of melatonin on U87MG malignant glioma cell line. Molecules 2018; [Crossref] [PubMed]