Osteopontin enhances cisplatin resistance of human A549 lung cancer cells via stimulating the PI3K signaling pathway and upregulating ERCC1 expression
Introduction
Lung cancer is one of the most serious malignant tumors with a high mortality rate worldwide (1). Despite the breakthroughs in molecular targeted cancer therapy in recent years, chemotherapy drugs remain one of the main treatment methods (2). Drug resistance is frequently developed in advanced lung cancer patients treated with chemotherapy, which greatly affects the effectiveness of chemotherapy as well as patients’ prognosis (3). The mechanism of cisplatin resistance in lung cancer involves a variety of complex signaling pathways, such as PI3K/AKT signaling pathway and excision repair cross complementary gene 1 (ERCC1) of nucleotide excision repair (NER) pathway (4). The PI3K (phosphoinositide 3-kinases)/AKT (protein kinase B) pathway activates its downstream regulator nuclear factor kappa B (NF-kB), thereby promoting the invasion, growth and metastasis of tumor cells (5). The over-activation of PI3K/AKT pathway may lead to tumor cell resistance to chemotherapeutic drug-induced apoptosis, and ultimately drug resistance (6). Platinum-based drugs exert their anti-tumor effects by impairing DNA synthesis and transcription, forming cisplatin-DNA adducts, and eventually inducing cell apoptosis (7). NER pathway is one of the major DNA repair pathways (8). The excision repair cross complementing-group 1 (ERCC1) gene plays an important role in NER-pathway due to its damage recognition and excision ability (9). Cisplatin-DNA adducts can by removed by NER, and thus overexpression of ERCC1 has been associated with cisplatin resistance in lung cancer (10).
Osteopontin (OPN) is a secretory phosphorylated glycoprotein that plays an important role in multiple biological processes, including bone resorption and mineralization, cell adhesion, migration, immune regulation, signal transduction, and inhibition of apoptosis (11). Through its interaction with integrin αvβ 3 and CD44 receptors, OPN activates multiple signaling pathways, alters cell adhesion, rearranges extracellular matrix, inhibits apoptosis, reconstructs cytoskeleton, and thus promotes invasion and metastasis of tumor cells (12). OPN has been detected in many tumors, including breast cancer (13), gastric cancer (14), liver cancer (15), head and neck tumor (16) and brain tumor (17), and may be a potential marker for evaluating tumor aggressiveness (18). OPN overexpression in colorectal cancer (19), ovarian cancer (20) and colorectal cancer (21) is closely related to tumor progression, tumor recurrence and patient survival. Moreover, the increase of OPN in the blood of cancer patients has been suggested as an independent indicator for metastasis and patient survival (12). Studies have shown that OPN overexpression can make cells more tumorigenic, invasive and metastatic, whereas down-regulation of OPN expression can inhibit such effects (22). Further studies have shown that OPN suppression can make these malignant and highly metastatic tumor cells become non-metastatic (23).
In this study, we constructed OPN overexpression and interference vectors, and evaluated the effects and regulatory mechanism of OPN in human non-small cell lung cancer (NSCLC) A549 cells. We further investigated if the regulatory role of OPN in tumor cell resistance to cisplatin involved the PI3K/AKT pathway and ERCC1 gene. The current study may provide novel therapeutic targets for the treatment of lung cancer, especially in cases of drug resistance to cisplatin-based chemotherapy.
Methods
Cell culture
Human A549 lung cancer cell line was purchased from Shanghai Branch of the Chinese Academy of Sciences. Cells were maintained in F12k medium (Gibco, Grand Island, NY, USA) containing 10% fetal bovine serum (Gibco), 100 U/mL penicillin and 100 µg/mL streptomycin (Keygene Biotech., Nanjing, China) at 37 °C, 5% CO2 in a cell incubator.
Construction of OPN expressing/silencing lentivirus
To construct OPN expressing lentiviral vector, the OPN cDNA sequences (NCBI number: NM_000582) was amplified by PCR and cloned into the plvx-puro vector with flanking XhoI and BamHI restriction sites following the Lentivector User Manual (Addgene, Cambridge, Mass., USA). To construct OPN silencing lentiviral vector, oligonucleotides coding for shOPN-forward: 5'-GATCCCTTTACAACAAATACCCAGATCTCGAGATCTGGGTATTTGTTGTAAAGTTTTTG-3', and shOPN-reverse: 5'-AATTCAAAAACTTTACAACAAATACCCAGATCTCGAGATCTGGGTATTTGTTGTAAAGG-3' were synthesized by Invitrogen Biotech. (Shanghai, China) and cloned into the vector pGreenPuro (Addgene). The constructed vectors were named OPN-plvxpuro and shOPN-pGreenpuro, respectively. Both constructs were confirmed by XhoI/BamHI digestion and sequencing. The plvx-puro vector was used as blank expression control. The lentiviral vector containing the non-silencing sequence (shNC-pGreenpuro) were used as blank interference control.
Cell transfection
A549 cells were cultured in 6-well plates and divided into normal control, blank interference control (shRNA-NC), interference (shRNA-OPN), blank expression control (NC), overexpression (OPN), control+cis-platinum (DDP), shRNA-NC+DDP, shRNA-OPN+DDP, OPN+DDP, and NC+DDP groups. Cells in shRNA-NC/shRNA-NC+DDP, shRNA-OPN/shRNA-OPN+DDP, NC/NC+DDP, and OPN/OPN+DDP were transfected with 20 nM of shNC-pGreenpuro, shOPN-pGreenpuro, plvxpuro, and OPN-plvxpuro, respectively using Lipofectamine 3000 transfection agent (Invitrogen) according to the manufacture’s instructions. After transfection, all DDP groups were cultured in medium supplemented with 5 µM DDP, and other groups were cultured in normal medium. Cells were incubated for 48 h and subjected to further experiments.
MTT assay
Cell proliferation was analyzed by MTT assay. Briefly, cells in different groups were collected and incubated with 20 µL of 5 mg/mL MTT (Sigma). After 4 h, cells were treated with 150 µL of dimethyl sulfoxide for 20 min. The optical density (OD) was detected with a microplate reader under the wavelength of 490 nm. The experiment was performed in triplicate.
Cell apoptosis assay
Cell apoptosis was detected by cell apoptosis assay kit (Multi Sceiences) using flow cytometry. Briefly, cells were resuspended in 1 × binding buffer, and stained with 5 µL of FITC Annexin V and 10 µL of PI in the dark for 10 min. The fluorescence of cells was then analyzed by a NovoCyteTM 2060R flow cytometer (Acea Biotech, Hangzhou, China) at the wavelength of 488 nm within 1 h.
Quantitative reverse transcription PCR (qRT-PCR)
mRNA expression was detected by qRT-PCR. Cells in different groups were collected at 48 h after transfection. Total RNA was extracted using the Trizol reagent (Kangwei Biotech., Beijing, China), and reverse transcribed into cDNA using HiFiScript cDNA kit (Kangwei Biotech.). Real-time PCR was performed using UltraSYBR mixture (Kangwei Biotech.) in a CFX Connect RT-PCR System (Bio-Rad, Shanghai, China). The following reactions conditions were used: 95 °C, 10 min, followed by 40 cycles of 95 °C, 10 s, 57–54 °C, 30 s, and 72 °C, 30 s. The following primers were synthesized by Invitrogen and used in the PCR: ERCC1-F: 5'-CCGCCAGCAAGGAAGAA-3', ERCC1-R: 5'-TGCCGAGGGCTCACAAT-3'; p-ERK1/2-F (extracellular signal-regulated kinases): 5'-TCCCAAATGCTGACTCCAA-3', p-ERK1/2-R: 5'-ACTCGGGTCGTAATACTGCTC-3', PI3K-F: 5'-CAATCCCAGGTGGAATGAA-3', PI3K-R: 5'-CAATCCCAGGTGGAATGAA-3', GAPDH-F: 5'-GAAGGTCGGAGTCAACGGAT-3', and GAPDH-R: 5'-CCTGGAAGATGGTGATGGG-3'. Data was analyzed using 2-ΔΔCt method. The relative expression was calculated using GAPDH as the internal control.
Western blot
Protein expression was determined by Western blot. Briefly, cells were collected at 48 h after transfection. Total protein was extracted using lysis buffer (Kangwei Biotech.) and quantified using BCA protein assay kit (Kangwei Biotech.). Equal aliquots (60 mg) of protein were separated by electrophoresis (10% SDS-PAGE) and transferred to polyvinylidene difluoride membranes. The membranes were then blocked with 5% skim milk for 30 min and incubated respectively with rabbit anti-p-ERK1/ERK2 polyclonal (phospho T202 + Y204, Abcam, 1:1,000), anti-PI3K monoclonal (Abcam, 1:1,000), anti-ERCC1 monoclonal (Abcam, 1:1,000) and mouse anti-GAPDH monoclonal (Zhongshan Gold Bridge Biotech., 1:3,000) overnight at 4 °C. The membrane was washed with PBS and incubated with HRP-conjugated goat anti-rabbit (Zhongshan Gold Bridge Biotech., 1:2,000) or anti-mouse (Zhongshan Gold Bridge Biotech., 1:2,000) IgG for 30 min at room temperature. The immunoreactivity was detected using an ECL Western blotting detection solution (Thermo Scientific, Shanghai, China). The relative expression of proteins was quantified using Quantity One software with GAPDH as the internal control.
Statistical analysis
All experiments were performed in triplicate. Data were expressed as mean ± standard deviation (SD) and analyzed by SPSS 19.0 software (IBM SPSS, Chicago, IL, USA). Differences among groups were compared using one-way ANOVA followed by post-hoc Tukey HSD test. Ratios were compared by Chi-square tests. P<0.05 was considered statistically significant.
Results
Verification of OPN overexpression and silencing
The constructed OPN-plvxpuro and shOPN-pGreenpuro were verified by enzymatic digestion and sequencing. Further, the expression of OPN mRNA in cells transfected with OPN-plvxpuro and shOPN-pGreenpuro was determined. Results showed that the expression of OPN mRNA was significantly decreased in shRNA-OPN group (P=0.038, Figure 1), but increased in OPN group (P=0.016) when compared with control group, suggesting that the transfection of constructs had successfully triggered overexpression and silencing of OPN gene. shRNA-NC and NC groups had similar OPN mRNA level to that in control group (P>0.05).
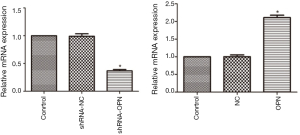
A549 cell viability was stimulated by OPN overexpression but reduced by OPN silencing
As shown in Figure 2, MTT assay demonstrated that cell viability in OPN group was significantly higher compared with control group (P=0.019). Cell viability in shRNA-OPN group was much lower than that in control group (P=0.041), suggesting that A549 cell viability was stimulated by OPN overexpression but reduced by OPN silencing. Cell viability in shRNA-NC and NC groups was close to that in control group. All DDP groups except OPN+DDP group showed significantly reduced cell viability (all P<0.05), indicating that OPN overexpression had reduced the inhibitory effects of DDP on cancer cells.
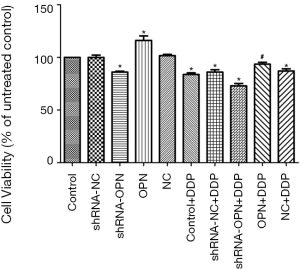
A549 cell apoptosis was decreased by OPN overexpression but increased by OPN silencing
Flow cytometry results showed that cell apoptosis rate was significantly lower in OPN group (P=0.020), but was markedly higher in shRNA-OPN compared with control group (P=0.029) than that in control group. OPN+DDP group had similar apoptosis rate to that in control group (P>0.05), whereas the other DDP groups exhibited significantly higher apoptosis rate (all P<0.05, Figure 3), suggesting that OPN overexpression had reversed the effects of DDP on cancer cells.
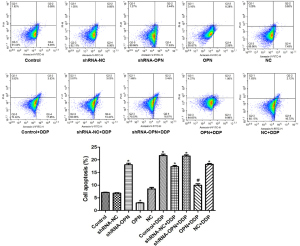
PI3K, p-ERK1/2 and ERCC1 expression was upregulated by OPN overexpression but down-regulated by OPN silencing
PI3K, p-ERK1/2 and ERCC1 protein and mRNA expression was analyzed by Western blot and qRT-PCR, respectively. As shown in Figure 4A, when compared with control group, the expression of PI3K, p-ERK1/2 and ERCC1 was obviously higher in OPN group (all P<0.05), but was much lower in shRNA-OPN group (all P<0.05). Among the 5 DDP groups, OPN+DDP had close PI3K, p-ERK1/2 and ERCC1 level to that in control group (P>0.05), whereas the other DDP groups had significantly lower protein expression (all P<0.05). Consistently, PI3K, p-ERK1/2 and ERCC1 mRNA expression showed similar trends among different groups (Figure 4B). These results showed that OPN had stimulated the PI3K signaling pathway and ERCC1 gene expression.
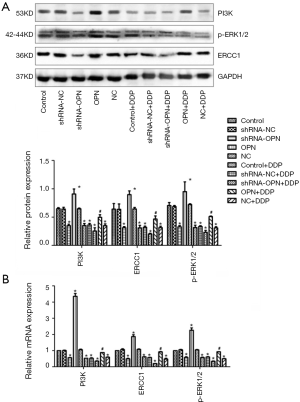
Discussion
Currently, cisplatin-based combination chemotherapy is the standard chemotherapy regimen. The clinical efficacy of cisplatin-based chemotherapy is between 15% and 40%, suggesting that many patients showed no responses to cisplatin or had developed resistance to the drug (24). Drug resistance of tumor cells has become one of the most prominent problems in chemotherapy of lung cancer. Cisplatin can cause DNA damage by forming adducts with DNA and thus initiate the process of DNA repair. Therefore, an increase in the rate of DNA adduct repair is an important mechanism of cisplatin resistance (25).
ERCC1 gene can be transcribed into a total of four different mRNAs with different molecular weights, but only the 1.1 kb mRNA can express a 39 x 104 protein with tolerance to platinum drugs (26). ERCC1 over-expression due to ERCC1 gene mutation has been suggested to be associated with tolerance to platinum-based chemotherapy in gastric cancer patients (27). Furthermore, ERCC1 mRNA expression is highly correlated with DNA repair ability of cells, and low expression of ERCC1 mRNA can increase the sensitivity of platinum-based chemotherapeutic drugs (28). Our results showed that the expression of ERCC1 protein was significantly down-regulated by the addition of cisplatin, indicating that cisplatin had decreased DNA repair ability and induced apoptosis of cancer cells. The activation of PI3K can induce anti-apoptosis effects and promote cell growth, which may eventually lead to drug resistance (29). Up-regulation of OPN expression can promote the growth of tumor cells via activating PI3K signaling pathway (30). Similarly, our results showed that overexpression of OPN had significantly increased PI3K expression and reduced cell apoptosis, whereas cisplatin treatment group and control group had similar PI3K expression and apoptosis rate. These results suggest that OPN may induce cisplatin resistance in NSCLC by activating PI3K signaling pathway. ERK1/2 are members of the mitogen-activated protein kinase family that can regulate the proliferation and apoptosis of cells. The phosphorylation of ERK1/2 activates their kinase activity and leads to phosphorylation of many downstream regulator involved in the process of cell proliferation (31). Studies have detected ERK1/2 overexpression plays a crucial role in regulating the biological behavior of tumor cells in lung cancer (32), breast cancer (33), prostate cancer (34) and several other malignancies. In this study, the expression of p-ERK1/2 was stimulated by OPN overexpression, but was reduced by shRNA-OPN. Moreover, the DDP had significantly inhibited the p-ERK1/2 expression, whereas OPN had greatly reversed such effects. Consistent with previous studies, our results suggested the OPN-induced cisplatin resistance in A549 cells may be mediated by the activity of ERK1/2.
In summary, this study has found that OPN stimulates the proliferation and reduces the apoptosis of human A549 lung cancer cells via activating the PI3K pathway and upregulating the ERCC1 expression. Moreover, OPN might also be involved in the drug resistance to DDP through regulating PI3K pathway and ERCC1 expression in A549 cells. Further experiments are needed to verify our findings in vivo.
Acknowledgments
Funding: The present research was funded by
Footnote
Conflicts of Interest: All authors have completed the ICMJE uniform disclosure form (available at http://dx.doi.org/10.21037/tcr.2020.03.60). The authors have no conflicts of interest to declare.
Ethical Statement: The authors are accountable for all aspects of the work in ensuring that questions related to the accuracy or integrity of any part of the work are appropriately investigated and resolved.
Open Access Statement: This is an Open Access article distributed in accordance with the Creative Commons Attribution-NonCommercial-NoDerivs 4.0 International License (CC BY-NC-ND 4.0), which permits the non-commercial replication and distribution of the article with the strict proviso that no changes or edits are made and the original work is properly cited (including links to both the formal publication through the relevant DOI and the license). See: https://creativecommons.org/licenses/by-nc-nd/4.0/.
References
- Bray F, Ferlay J, Soerjomataram I, et al. Global cancer statistics 2018: GLOBOCAN estimates of incidence and mortality worldwide for 36 cancers in 185 countries. CA Cancer J Clin 2018;68:394-424. [Crossref] [PubMed]
- Hu Q, Sun W, Wang C, et al. Recent advances of cocktail chemotherapy by combination drug delivery systems. Adv Drug Deliv Rev 2016;98:19-34. [Crossref] [PubMed]
- Bonanno L, Favaretto A, Rosell R. Platinum drugs and DNA repair mechanisms in lung cancer. Anticancer Res 2014;34:493-501. [PubMed]
- Köberle B, Tomicic MT, Usanova S, et al. Cisplatin resistance: preclinical findings and clinical implications. Biochim Biophys Acta 2010;1806:172-82.
- Ghoneum A, Said N. PI3K-AKT-mTOR and NFκB Pathways in Ovarian Cancer: Implications for Targeted Therapeutics. Cancers (Basel) 2019;11:949. [Crossref] [PubMed]
- Brown KK, Toker A. The phosphoinositide 3-kinase pathway and therapy resistance in cancer. F1000Prime Rep 2015;7:13. [Crossref] [PubMed]
- Dasari S, Tchounwou PB. Cisplatin in cancer therapy: molecular mechanisms of action. Eur J Pharmacol 2014;740:364-78. [Crossref] [PubMed]
- Kusakabe M, Onishi Y, Tada H, et al. Mechanism and regulation of DNA damage recognition in nucleotide excision repair. Genes Environ 2019;41:2. [Crossref] [PubMed]
- Friboulet L. ERCC1 isoform expression and DNA repair in non-small-cell lung cancer. N Engl J Med 2013;368:1101-10. [Crossref] [PubMed]
- Muallem MZ, Braicu I, Nassir M, et al. ERCC1 expression as a predictor of resistance to platinum-based chemotherapy in primary ovarian cancer. Anticancer Res 2014;34:393-9. [PubMed]
- Hao C, Cui YX, Owen S, et al. Human osteopontin: Potential clinical applications in cancer Int J Mol Med 2017;39:1327-37. (Review). [Crossref] [PubMed]
- Zhao H, Chen Q, Alam A, et al. The role of osteopontin in the progression of solid organ tumour. Cell Death Dis 2018;9:356. [Crossref] [PubMed]
- Gao YL, Xing LQ, Ren TJ, et al. The expression of osteopontin in breast cancer tissue and its relationship with p21ras and CD44V6 expression. Eur J Gynaecol Oncol 2016;37:41-7. [PubMed]
- Cao DX, Li ZJ, Jiang XO, et al. Osteopontin as potential biomarker and therapeutic target in gastric and liver cancers. World J Gastroenterol 2012;18:3923-30. [Crossref] [PubMed]
- Brůha R, Vitek L, Smid V. Osteopontin – A potential biomarker of advanced liver disease. Ann Hepatol 2020; [Crossref] [PubMed]
- Snitcovsky I, Leitão GM, Pasini FS, et al. Plasma osteopontin levels in patients with head and neck cancer undergoing chemoradiotherapy. Arch Otolaryngol Head Neck Surg 2009;135:807-11. [Crossref] [PubMed]
- Jan HJ, Lee CC, Shih YL, et al. Osteopontin regulates human glioma cell invasiveness and tumor growth in mice. Neuro Oncol 2010;12:58-70. [Crossref] [PubMed]
- Wei R, Wong JPC, Kwok HF. Osteopontin - a promising biomarker for cancer therapy. J Cancer 2017;8:2173-83. [Crossref] [PubMed]
- Cheng Y, Wen G, Sun Y, et al. Osteopontin Promotes Colorectal Cancer Cell Invasion and the Stem Cell-Like Properties through the PI3K-AKT-GSK/3β-β/Catenin Pathway. Med Sci Monit 2019;25:3014-25. [Crossref] [PubMed]
- Song G, Cai QF, Mao YB, et al. Osteopontin promotes ovarian cancer progression and cell survival and increases HIF-1alpha expression through the PI3-K/Akt pathway. Cancer Sci 2008;99:1901-7. [PubMed]
- Ng L, Wan T, Chow A, et al. Osteopontin overexpression induced tumor progression and chemoresistance to oxaliplatin through induction of stem-like properties in human colorectal cancer. Stem Cells Int 2015;2015:247892.
- Sheng XJ, Zhou DM, Liu Q, et al. BRMS1 inhibits expression of NF-kappaB subunit p65, uPA and OPN in ovarian cancer cells. Eur J Gynaecol Oncol 2014;35:236-42. [PubMed]
- Shevde LA, Samant RS, Paik JC, et al. Osteopontin knockdown suppresses tumorigenicity of human metastatic breast carcinoma, MDA-MB-435. Clin Exp Metastasis 2006;23:123-33. [Crossref] [PubMed]
- Makovec T. Cisplatin and beyond: molecular mechanisms of action and drug resistance development in cancer chemotherapy. Radiol Oncol 2019;53:148-58. [Crossref] [PubMed]
- Rocha CRR, Silva MM, Quinet A, et al. DNA repair pathways and cisplatin resistance: an intimate relationship. Clinics (Sao Paulo) 2018;73:e478s. [Crossref] [PubMed]
- Lafuente-Sanchis A, Zúñiga Á, Galbis JM, et al. Prognostic value of ERCC1, RRM1, BRCA1 and SETDB1 in early stage of non-small cell lung cancer. Clin Transl Oncol 2016;18:798-804. [Crossref] [PubMed]
- Wan J, Chao L, Lee AC, et al. Higher expression of ERCC1 may be associated with resistance to adjuvant platinum-based chemotherapy in gastric cancer. Cancer Invest 2017;35:85-91. [Crossref] [PubMed]
- Chebouti I, Kuhlmann J D, Buderath P, et al. ERCC1-expressing circulating tumor cells as a potential diagnostic tool for monitoring response to platinum-based chemotherapy and for predicting post-therapeutic outcome of ovarian cancer. Oncotarget 2017;8:24303-13. [Crossref] [PubMed]
- West KA, Castillo SS, Dennis PA. Activation of the PI3K/Akt pathway and chemotherapeutic resistance. Drug Resist Updat 2002;5:234-48. [Crossref] [PubMed]
- Liang J, Xu L, Zhou F, et al. MALAT1/miR-127-5p regulates osteopontin (OPN)-mediated proliferation of human chondrocytes through PI3K/Akt pathway. J Cell Biochem 2018;119:431-9. [Crossref] [PubMed]
- Roux PP, Blenis J. ERK and p38 MAPK-activated protein kinases: a family of protein kinases with diverse biological functions. Microbiol Mol Biol Rev 2004;68:320-44. [Crossref] [PubMed]
- Vicent S, López-Picazo JM, Toledo G, et al. ERK1/2 is activated in non-small-cell lung cancer and associated with advanced tumours. Br J Cancer 2004;90:1047-52. [Crossref] [PubMed]
- Zhang M, Song S, Yi Z, et al. Human biliverdin reductase promotes EMT through the ERK1/2 signal pathway in breast cancer. Eur J Pharmacol 2016;788:45-53. [Crossref] [PubMed]
- Jin F, Irshad S, Yu W, et al. ERK and AKT signaling drive MED1 overexpression in prostate cancer in association with elevated proliferation and tumorigenicity. Mol Cancer Res 2013;11:736-47. [Crossref] [PubMed]