Proton beam radiation therapy of prostate cancer - history, results, and future directions
Introduction
Prostate cancer continues to present a major oncologic dilemma for the developed world. In the United States there were an estimated 240,000 new cases diagnosed in 2011, with approximately 33,000 deaths from this disease (1). Prostate cancer is the second leading cause of cancer deaths among American men and accounts for approximately 10% of all cancer related deaths in men. A similar incidence and death rate is seen in Western Europe, with the lowest reported incidence being in Eastern/Southern Asia. Beginning in the early 1990’s the discovery and use of Prostate Specific Antigen (PSA) as a screening tool has led to both an increase in the number of cases being diagnosed and a decrease in the proportion of men being diagnosed with advanced disease. This encouraging trend towards diagnosis with organ-confined disease has prompted the development and refinement of treatment methods directed at the prostate in the entirely reasonable hope of providing long-term disease free survival and cure.
From the standpoint of radiotherapy virtually all technical advances in prostate cancer treatment have been implemented to reduce normal tissue toxicity by limiting the volume of adjacent bladder and rectum which receive moderate to high doses of radiation. A direct consequence of this improvement in dose conformity has been dose escalation (2), a concept which has been tested and confirmed in one proton beam-based prospective randomized trial.
The unique physical properties inherent in proton beams makes them particularly attractive to the radiation oncologist, for they permit a reduction in “integral dose” (defined as the total radiation dose given to the patient) over and above anything which can be achieved with photon-based external beam treatment systems (3-5).
Initial proton beam treatment results
The ability to use proton therapy to treat deep organs was, and remains, greatly dependent on the concurrent development and refinement of cross-sectional imaging technology [CT, MRI] and modern computers, hence it is not surprising that proton beam therapy of prostate cancer did not commence until the late 1970’s. Beginning in 1977, Shipley and associates at the Massachusetts General Hospital [MGH] initiated a Phase I trial in which proton beam radiotherapy was used to give a boost dose to patients with locally advanced disease that were also receiving photon radiotherapy. At that time, this boost dose was felt to be over and above what could be safely given with existing 2-dimensional photon technology. Seventeen patients with stage T2-T4 disease received a perineally-directed proton beam boost of 20-26 GyE (given at a rate of 1.8-2 GyE/day) following treatment to the prostate and pelvis to a dose of 50.4 Gy with 10 MV photons given via a four-field box approach. A perineal approach was chosen because this was the only anatomical pathway that allowed the 160 MeV proton beam generated by the Harvard Cyclotron to reliably encompass the entire prostate gland. Acutely, the treatment was well tolerated and after a follow up period ranging from 12-27 months no severe late rectal reactions were noted (6).
These favorable toxicity results led directly to the initiation of a prospective randomized trial designed to test the benefits of proton beam dose escalation in patients with locally advanced disease. Patients with stage T3-T4 tumors were chosen as it was felt that this group stood to gain the most benefit from high doses. All patients received 50.4 Gy to the prostate and pelvis with megavoltage photons, administered via a four-field box-technique. They were then randomly assigned to receive either an additional 16.8 Gy of photons (for a total prostate dose of 67.2 Gy) or 25.2 GyE of protons for a total prostate dose of 75.6 Gy. Adjuvant hormonal therapy was not permitted. The limited availability of the Harvard Cyclotron affected patient accrual; nonetheless, two hundred and two patients were eventually enrolled, with one hundred and three being treated in the high dose arm and ninety nine in the standard dose arm.
With a median follow up of 61 months there were no differences seen in overall survival, disease-specific survival, total relapse-free survival, or local control between the arms. Patients with high-grade tumors who were treated on the high dose arm did experience a trend towards improvement in local control at five and eight years (92% and 77% vs. 80% and 60%, P=0.089). Patients whose digital rectal exams normalized following treatment and who underwent subsequent prostate biopsy revealed a lower positive biopsy rate in the high dose arm (28% vs. 45%) and, perhaps most surprisingly, the local control rates for patients with Gleason grade 4-5 tumors (57 patients total) were significantly better at five and eight years in the high dose patients (94% & 84% vs. 68% & 19%, P=0.001). High dose treatment was associated with an increase in late grade 1-2 rectal bleeding (32% vs. 12%, P=0.02) (7).
These results have been erroneously cited by some as evidence that proton-beam dose escalation is of doubtful utility (8). However, it must be noted that the patients treated in this trial were at a high risk of not only local failure but of distant failure and therefore it is not surprising that overall survival was unaffected. In addition, patients with these adverse characteristics would not, if diagnosed today, receive radiotherapy as monotherapy and instead would be treated with a multi-modality approach (9-12). What the trial did demonstrate is that (I) high dose radiotherapy did decrease local failure, and this decrease was most profound in those patients with the most aggressive tumors and (II) Dose-escalation by means of a perineal proton beam (an approach which has largely been abandoned today as higher energy proton beams have become available) can be performed safely with acceptable toxicity.
The completion in 1990 of the world’s first hospital-based proton treatment center at Loma Linda University Medical Center [LLUMC] marked the beginning of a transition in proton beam therapy from the research laboratory setting to clinical radiation oncology (13). Beginning in late 1991 prostate patients at LLUMC were treated on a clinical trial who’s goal was to confirm the efficacy and toxicity data generated at MGH. Between December 1991 and December 1995 643 patients were treated to total prostate radiation doses of 74-75 GyE. Patients who were deemed to be at a low risk for occult nodal metastasis were treated with lateral proton beams alone while those who were felt to benefit from elective nodal radiation received 45 Gy to the pelvis with 18-23 MV photons delivered via a multifield 3-D conformal technique. Patient characteristics are shown in Table 1.
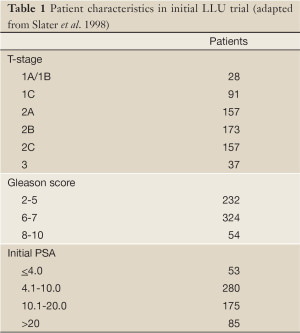
Full table
With a median follow up of 43 months, the overall biochemical disease-free survival [bNED] rate was 79% as per the American Society for Therapeutic Radiology and Oncology [ASTRO] definition of three successively rising PSA values above a nadir equating to biochemical failure. The risk of biochemical failure was strongly dependent on the pre-treatment PSA with five-year bNED survival rates varying from 53% in patients with pre-treatment PSA’s of 20-50 to 100% with PSA’s of <4.1. BNED survival was also significantly influenced by post-treatment PSA nadir. A multi-variant analysis of failure predictors demonstrated that initial stage, PSA, and Gleason Score were all strong predictors of biochemical failure at five years (Table 2). Acute toxicity was minimal and all patients completed the prescribed course of radiotherapy. Proctitis remained the most common late toxicity with Grade 2 proctitis occurring in 21% of patients at three years; for the majority of patients this represented a single episode of rectal bleeding. No > Grade 3 GI toxicity was seen. Grade 2 GU toxicity (primarily gross hematuria) was seen in 5.4% of patients at three years, with two patients developing Grade 3 bladder toxicity. No significant difference in late toxicity was seen between those patients treated with protons alone and those receiving pelvic x-ray therapy (14).
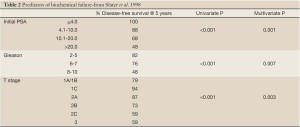
Full table
An update of the initial LLUMC experience was published in 2004. This study encompassed 1,255 patients with stage T1-T3 disease who were treated with proton beam radiotherapy alone (i.e., no prior or concurrent hormonal therapy) to a dose of 74-75 GyE. As was seen in the earlier trial initial PSA, Gleason Grade, and PSA nadir were all strong predictors of bNED survival. Treatment continued to be well tolerated with rates of RTOG Grade >3 GI/GU late morbidity of <1% (15).
PROG/ACR95-09 randomized dose-escalation trial
Beginning in 1996, LLUMC and MGH embarked on the Proton Radiation Oncology Group/American College of Radiology [PROG/ACR] 95-09 trial, a prospective, randomized dose-escalation study for patients with organ-confined prostate cancer. This study was designed to test the hypothesis that a dose escalation from 70.2 to 79.2 GyE would result in a statistically significant decrease in local failure, biochemical failure, and overall survival. Eligibility criteria included stage T1b-T2b disease (as per the 1992 American Joint Committee on Cancer staging system), a PSA of <15 ng/mL, and no evidence of metastatic disease on imaging studies (bone scan, abdominal-pelvic CT scan). All Gleason scores were allowed, but no prior or concurrent androgen-deprivation therapy was permitted. Pre-treatment patient characteristics are shown in Table 3.
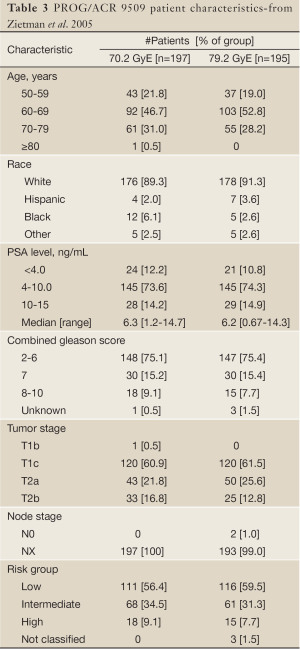
Full table
Patients were randomly assigned to receive a total prostate dose of 70.2 or 79.2 GyE. Radiotherapy was administered sequentially in two phases. In Phase I, conformal proton beams were used to treat the prostate alone. Depending on randomization either 19.8 or 28.8 GyE in 11 or 16 fractions was delivered. The clinical target volume [CTV] was the prostate plus a 5 mm margin. Beam arrangement was facility dependent with patients at LLUMC being treated with lateral proton beams of 225-250 MeV energy, while at MGH a perineal 160 MeV proton beam was employed. Before each proton beam treatment session a water balloon was inserted into the rectum and inflated with 100 mL of saline; this served the dual purpose of distending the rectum lumen to decrease the volume of rectum receiving any radiation and minimizing prostate motion.
In the second phase of treatment all patients received 50.4 Gy of photons given in twenty-eight 1.8 Gy fractions. The CTV was the prostate and seminal vesicles. No effort was made to include the pelvic lymphatics. Three-dimensional planning was used on all patients and photon energies of 10-23 MV were employed. The use of photons for a portion of the treatment was done solely to allow both institutions to participate in this trial, for at the time the trial commenced MGH patients were still restricted to treatment at the Harvard Cyclotron Laboratory and the limited throughput of that facility meant that the most efficient use of protons was as a boost and not as monotherapy. A total of 393 patients were randomized between January 1996 and December 1999.
The results of the trial were initially published in 2005 (16), with an update in 2010. At a median follow-up of 8.9 years there is a persistent and statistically significant increase in biochemical freedom from relapse amongst patients randomized to the high dose arm (Figure 1). This difference was seen when using both the ASTRO and the more recent Phoenix definition (17) (in which biochemical failure = a PSA elevation of >2 ng/mL above a nadir). Subgroup analysis showed a particularly strong benefit in 10-year bNED survival amongst the “low risk” patients (defined as PSA <10 ng/mL, and Gleason score <7 and stage < T2b), with 92.2% of high dose patients being disease free vs. 78.8% for standard dose (P=0.0001). A strong trend towards a similar finding was seen in the intermediate risk patients but this has not reached statistical significance (Figure 2). In addition, patients in the standard dose arm are twice as likely to have been started on androgen deprivation therapy as high dose patients (22 vs. 11, P=0.47) with such treatment usually being initiated due to a rising PSA. To date, there is no difference in overall survival between the arms (18).
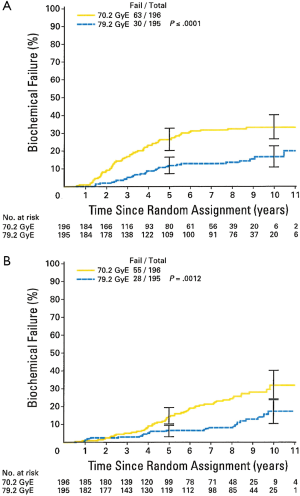
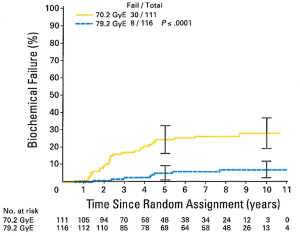
As was seen in the previously reported proton trials treatment was well tolerated. Only 2% of patients in both arms have experienced late GU toxicities of Grade >3 and 1% have experienced late GI toxicity of Grade >3. Interestingly, as opposed to what has been reported in some photon-based randomized dose escalation trials high dose radiotherapy delivered via a conformal proton beam boost did not result in an increase in late Grade >3 GI morbidity amongst the high dose patients (Table 4). This encouraging finding has been confirmed by a patient-reported sensitive Quality of Life instrument which did not report any greater morbidity than the physician-reported scores, and which revealed equal and high satisfaction with quality of life between both arms (19).
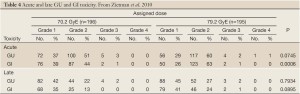
Full table
Thus, the PROG/ACR 9509 trial provides “Level One” evidence verifying the importance of radiation dose-escalation in organ confined prostate cancer and while this study was not designed to directly compare the efficacy of conformal proton beam radiotherapy against other conformal techniques or modalities it does demonstrate that conformal proton beam radiotherapy is an effective treatment for this disease, with minimal risk of experiencing severe treatment-induced toxicity.
University of Florida experience
The University of Florida Proton Therapy Institute opened in the summer of 2006 with prostate cancer treatment commencing at that time. From August, 2006 to October 2007 patients were treated on one of three prospective trials: 78 GyE/39 fractions for low-risk disease, dose escalation from 78-82 GyE for intermediate-risk disease, and 78 GyE with concomitant taxotere, followed by androgen-deprivation therapy, for high-risk disease. Preliminary GI and GU toxicity data was reported in 2010 with a minimum of two year follow up. Forty-two percent of the patients experienced Grade 2+ GU symptomatology requiring management after treatment, including four transient Grade 3 symptoms (all of which occurred in patients who required medical or surgical management of GU symptoms prior to radiotherapy). The overwhelming majority of Grade 2 symptoms (98%) were retentive symptoms requiring treatment with alpha-blockers. Multivariate analysis suggested that Grade 2+ GU toxicities were correlated with pre-treatment prostatitis, pre-treatment International Prostate Symptom Score [IPSS] score and, as time progressed, with patient age and pre-treatment GU symptom management. This strongly suggests that the predominant predictors of early GU toxicity were pre-treatment clinical factors.
GI toxicities were considerably less common, 10% of the patients experiencing a cumulative incidence of Grade 2+ GI toxicities over the first two years post-treatment, including a single Grade 3 toxicity. Univariate analysis revealed a significant correlation between Grade 2 or higher GI toxicity and the percentage of rectal wall receiving radiation doses from 40-80 GyE, and the percentage of rectum receiving 10-80 GyE, while multivariate analysis revealed the rectal wall V70 correlated with the cumulative incidence of Grade 2+ rectal bleeding and/or proctitis at 24 months. The authors concluded that treatment was well tolerated with minimal and acceptable GI/GU toxicity, again mirroring the results from other proton centers (20).
ACR 0312 trial
Following the completion of patient accrual to the PROG/ACR9509 randomized trial, LLUMC and MGH opened a Phase II dose-escalation study designed to determine the toxicity and efficacy of proton-beam based dose escalation in patients with organ-confined disease. The ACR 0312 trial delivered a total dose of 82 GyE/41 fractions to the prostate, with the initial 50 GyE also including the caudal 2 cm of the seminal vesicles. PTV volumes were identical to those used in the PROG 9509 patients. The trial enrolled eighty-five patients who were treated between May 2003 and March 2006. The rate of acute GI/GU > Grade 3 complications were 1%. With a median follow up of 31.6 months six patients have developed a late Grade 3 GI/GU toxicity with one additional patient developing Grade 4 toxicity. The median time to Grade 3+ toxicity was 9.5 months with an estimated rate of Grade 3+ toxicity at eighteen months of 6%. Dose-Volume Histogram [DVH] analysis of the radiation dose to the anterior rectal wall failed to reveal a demonstrable association between dose to various volumes of the anterior wall and the risk of subsequently developing a Grade 2+ late rectal toxicity. The authors noted that the observed late morbidities compare favorably with that reported in IMRT dose-escalation studies, but that the dose of 82 GyE/41 fractions may represent the safe limit of what can be delivered with passive-scattered proton beams. They speculated that further dose-escalation should be possible with the forthcoming implementation of intensity modulated proton beams and real-time image-guided proton treatment delivery (21).
Japan
The Hyogo Ion Beam Medical Center began treating prostate patients with proton radiation in April 2001. Between 2001-2002 a series of Phase I-II protocols were performed to verify treatment techniques and assess toxicity. Once these revealed minimal toxicity proton beam therapy passed into general clinical use (22). In 2003-2004, 287 patients with stage T1-T4 N0 M0 prostate cancer were treated with lateral proton beams to a dose of 74 GyE in 37 fractions. Planning margins were similar to those used at the US proton centers, although a rectal balloon was not used. Patient characteristics are shown in Table 5 (23). Seventy-one percent of the patients also received androgen-deprivation therapy.
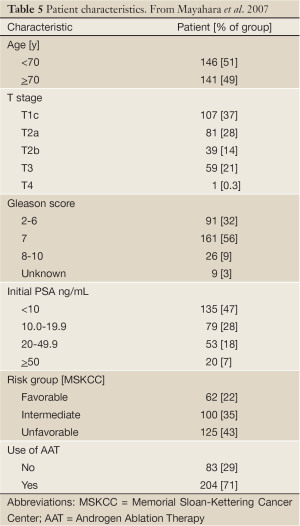
Full table
The observed morbidities are shown in Table 6. Mirroring the US experience, Grade 3 GU toxicities were extremely rare, and no Grade 4 events occurred. On Univariate analysis CTV size and patient age were significantly associated with a greater incidence of Grade >2 GU morbidity. Multivariate analysis confirmed that large CTV’s [P=0.001] and the use of androgen suppression therapy [P=0.017] independently predicted acute GU Grade 2-3 morbidity. These acute toxicities were comparable to those seen in published IMRT, 3-D conformal, and Brachytherapy series.
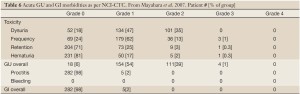
Full table
Protons vs. IMRT
In a widely quoted 2012 study, Sheets and colleagues at the University of North Carolina performed a comparison of prostate cancer patients treated with IMRT to those receiving 3-D conformal radiation therapy or proton beam treatment. The study reviewed patients from the SEER and Medicare databases who were treated between 2000 and 2007. Disease-free status was assessed by the need for additional cancer therapy and late morbidity was assessed by the need for additional diagnostic and/or therapeutic procedures to address radiation-induced problems.
The authors concluded that while IMRT was superior to 3-D conformal radiation therapy in terms of disease-free status and late morbidity, proton beam therapy carried with it (as compared to IM RT) an increased risk of late gastrointestinal morbidity for no therapeutic gain (24).
I believe that there are substantial methodological flaws in the study, which could easily explain the observed results:
(I) The authors made no attempt to account for likely 10-15% difference in radiation dose between the proton and IMRT patients. During the time period encompassed by this study, the “typical” IMRT radiation dose was between 70-74 Gray, the largest series of randomized data favoring dose escalation in prostate cancer was not published until 2005 and even after this paper was published it still took several years for the radiation oncology community to accept the increased external beam radiation dose of 79-81 Gray as “standard”. In contrast, all the proton patients analyzed in this trial were treated at a single SEER institution, and all received a minimum radiation dose of 79.2 Gray, with many receiving 80-81 Gray. As has been previously published late gastrointestinal morbidity is highly dependent upon both total radiation dose and normal-organ delineation (13,25), so the difference in late gastrointestinal morbidity between the proton beam and IMRT patients can be easily explained simply by the higher radiation dose routinely given to the proton beam patients.
(II) In contrast to the situation prevalent in the community, all of the proton beam patients were treated on protocols that called for close and regular follow-up with particular attention being paid to gastrointestinal issues, and which mandated gastrointestinal evaluation for any late gastrointestinal complaints. Since this study did not analyze severity of gastrointestinal issues but only the need for additional diagnostic and/or therapeutic procedures, this inherent bias in the proton patients towards protocol-mandated gastrointestinal referral can explain the greater number of gastrointestinal event seen in the proton beam patients.
(III) No attempt was made by the authors to analyze any potential differences in prostate gland and rectal wall coverage between the IMRT and Proton patients via a dose-volume-histogram analysis. Indeed, the authors fail to comment on any of the technical aspects of the two different types of radiotherapy analyzed. Were identical treatment margins used on all patients? How was the dose proscribed? What immobilization, if any was used? Was image-guidance employed and if so what type? When one considers the heterogeneous nature of the IMRT patients who were treated at multiple facilities versus the homogeneous nature of the proton patients, all of whom were treated at a single center with well-defined and adhered to protocols for dose prescription, patient immobilization, and daily positioning, these technical factors become even more important as they could easily in and of themselves result in the difference in morbidity noted between the two groups.
All this serves to illustrate the risks and potential inaccuracies inherent in attempting to use large patient registries to perform a detailed data analysis. Unfortunately, papers such as the Sheets paper, once published, are often quoted as having “proved” a particular point when in fact they have done nothing substantive to settle the issue. The definitive way to answer the protons vs. IMRT question would be to perform a prospective randomized trial but this is no more likely to occur than were randomized 3-D conformal X-ray vs. IMRT trials when the latter technology was first being introduced, and for the same reason-randomizing patients to potentially receive more of a toxic substance (radiation) whose toxicity is beyond questioning and which is of no benefit whatsoever to the patient is ethically suspect and in all likelihood such a trial would, if attempted, fail to reach its accrual goal (26).
Hypofractionation
Modern radiobiologic theory predicts that prostate cancer has a low “alpha/beta ratio”. This is a numeric description of the sensitivity of a particular tissue to radiation fraction size. For example, tissues with a low alpha/beta ratio are more sensitive to changes in fraction size than those with a high alpha-beta ratio, with most estimates for prostate cancer cells being in the range of 1.5-2.0 (27). This is substantially lower than the alpha/beta ratio of 3-4 that has been assumed for late bladder/rectal toxicity. This difference in alpha/beta ratios implies that prostate cancer cells are more sensitive to changes in radiation fraction size than those of the bladder or rectum, meaning that by increasing the daily fraction size and reducing the total radiation dose one can potentially shorten the overall treatment time without compromising tumor control and without increasing the risk of incurring a late GI/GU injury.
Hypofractionation has a long-established history in proton beam therapy, and is now routinely used in proton beam treatment of ocular melanomas, intracranial metastasis, arterial-venous malformations (28), lung cancer (29), and breast cancer (30). It also is being actively investigated in prostate cancer, although to date this investigation has employed primarily IMRT-based approaches (31-34). There is an emerging body of data supporting its safety and efficacy in this setting to the point that at least one prominent radiation biologist has declared that hypofractionation should be considered the treatment of choice for prostate cancer (35).
At the time of this writing there are at least four hypofractionated conformal proton beam treatment protocols actively accruing patients in the USA. At LLUMC, a Phase I-II trial of 60 GyE/20 fractions (which is designed to be isoeffective with 81 GyE/ 45 fractions, if one assumes an alpha/beta ratio of 1.5 for prostate cancer) began accruing patients in 2009. Eligibility is limited to “low risk” patients (PSA <10 ng/mL, Gleason <7, and Stage < T2b). Preliminary analysis indicates that treatment is well tolerated with no patient (n=50) experiencing a Grade >3 acute GI/GU complication. Post-treatment PSA decreases are consistent with expectations. At the University of Florida hypofractionation is being investigated in a similar protocol in which patients with low to intermediate-risk prostate cancer are treated on a 5-week hypofractionated schedule to a total dose of 70 GyE/28 fractions for low-risk patients, and 72.5 GyE/29 fractions for intermediate risk patients. The Proton Collaborative Group is performing a Phase III randomized trial of standard vs. hypofractionated proton radiation in low-risk patients, while the University of Pennsylvania is performing a feasibility trial of “mildly hypofractionated” proton radiation therapy or IMRT in intermediate-risk patients.
Proton treatment-summary
The published peer-reviewed data conclusively demonstrates that conformal proton beam radiotherapy is extremely well tolerated and can produce bNED survival rates equivalent to other modern radiotherapy modalities, and to radical prostatectomy. Conformal proton beam dose-escalation has been tested in a prospective randomized trial and has been shown to improve bNED survival without [as opposed to what has been seen in some x-ray based trials (36)] concurrently increasing the risk of late Grade >3 GI/GU morbidity. However, attempts to escalate dose to 82 GyE have been met with a substantial increase in late GI morbidity; this may reflect the “limit” beyond which treatment with passive-scattered beams and their attendant substantial penumbra may not be safely possible, although it is likely that the pending introduction of intensity-modulated proton therapy [IMPT] via active beam scanning and the implementation of novel image-guided techniques will permit further increases in dose. Hypofractionation is currently being tested in protocols at several proton centers and preliminary data on the safety and efficacy of this technique will be available within the next 12-18 months.
Future directions
Prostate cancer is an excellent site in which to test and perfect the implementation of new treatment techniques and dose-fractionation schedules. Ongoing technical advances in proton beam therapy will lead to further dose-specificity within the target organ and a further reduction in normal tissue radiation dose. Development of these techniques, including IMPT and real-time particle beam IGRT, will require their testing in a large number of patients who have similar disease characteristics and anatomic constraints. Prostate cancer represents an excellent “test bed” for these important developments. It is an extremely common disease so large numbers of potential patients exist and, as opposed to some other common tumors (most notably lung cancer) it is typically diagnosed while confined to its organ of origin so that treated patients are likely to live for the many years post treatment required to perform a comprehensive analysis of late effects. Organ motion is minimal, which aids in the development of beam-scanning techniques that are inherently more sensitive to target motion than passive-scattered arrangements. That fact that tumor response can be assessed biochemically as opposed to clinically or radiologically means that the effects of alterations in treatment techniques on tumor can be analyzed (and potentially adjusted or even abandoned) far more rapidly than when less exacting measures are available. Lastly, in contrast to other sites like the base of skull, the prostate is adjacent to only two critical organs about which a good deal is already known concerning dose-volume effects and their impact on acute and late morbidity, thereby providing for a more accurate extrapolation of the effects of any potential treatment alterations than would be true of other, less frequently treated sites.
One of the often-voiced complaints about proton beam treatment is the cost of providing this therapy. This concern is commonly raised whenever any new treatment technology or, for that matter, any new technology, is introduced into society. In the health care arena, new technology is increasingly being met with the demand that the new method be subjected to randomized trials vs. existing treatment methods before the medical community and health care payers accept the new method.
This clamor for randomized data is not new, nor is it confined to the introduction of proton beam treatment. It is imperative to remember that virtually all other advancements in radiotherapy treatment technology, including the widespread embracement of IMRT, have not occurred only after this technology was first tested in prospective trials but solely because this technology promised a higher degree of dose conformality than its contemporaries. When considered from this perspective, proton beam therapy is best viewed as simply a further large step along the same road of technological advancement that has been followed diligently by radiation oncologists for the last century. A randomized proton/IMRT trial would expose (literally) one group of patients to an integral dose 3-4 times greater than the other, with no expected gain in terms of disease control. Attempts to convince educated patients to participate in such a study in meaningful numbers will be difficult at best may well prove to be impossible.
It is also quite likely that the cost of proton beam radiation therapy (again, mirroring the cost of any new technology, with computers being a prime example) will inevitably decline as demand for this technology fosters the continuing development of newer, less expensive treatment units. Once the cost of proton beam treatment approximates that of IMRT arguments over relative efficacy will in all likelihood come to an abrupt end. In order for proton beam treatment to achieve this goal it has to be used for treatment of common cancers like prostate cancer. Again, this pathway is not new, and it simply follows the trail already blazed by other technologies, including IMRT.
The prostate represents perhaps the ideal proving ground for proton beam treatment. Rather than discourage its use on prostate cancer I believe that its use should be encouraged. The techniques perfected and lessons learned will serve to benefit all patients, including those treated with other radiotherapy modalities, and will add invaluable data to the widespread clinical implementation of proton beam radiotherapy.
Acknowledgments
Funding: None.
Footnote
Provenance and Peer Review: This article was commissioned by the Guest Editors (Huan Giap and Eric Y Chuang) for the series “Particle Beam Therapy I” published in Translational Cancer Research. The article has undergone external peer review.
Conflicts of Interest: The author has completed the ICMJE uniform disclosure form (available at http://dx.doi.org/10.3978/j.issn.2218-676X.2012.10.06). The series “Particle Beam Therapy I” was commissioned by the editorial office without any funding or sponsorship. The author has no other conflicts of interest to declare.
Ethical Statement: The author is accountable for all aspects of the work in ensuring that questions related to the accuracy or integrity of any part of the work are appropriately investigated and resolved.
Open Access Statement: This is an Open Access article distributed in accordance with the Creative Commons Attribution-NonCommercial-NoDerivs 4.0 International License (CC BY-NC-ND 4.0), which permits the non-commercial replication and distribution of the article with the strict proviso that no changes or edits are made and the original work is properly cited (including links to both the formal publication through the relevant DOI and the license). See: https://creativecommons.org/licenses/by-nc-nd/4.0/.
References
- Mohler J, Bahnson RR, Boston B, et al. NCCN clinical practice guidelines in oncology: prostate cancer. J Natl Compr Canc Netw 2010;8:162-200. [PubMed]
- Pickles T, Pollack A. The case for dose escalation versus adjuvant androgen deprivation therapy for intermediate risk prostate cancer. Can J Urol 2006;13:68-71. [PubMed]
- Archambeau JO, Bennett GW, Levine GS, et al. Proton radiation therapy. Radiology 1974;110:445-57. [PubMed]
- Archambeau JO, Bennett GW, Chen ST. Potential of proton beams for total nodal irradiation. Acta Radiol Ther Phys Biol 1974;13:393-401. [PubMed]
- Loeffler JS, Smith AR, Suit HD. The potential role of proton beams in radiation oncology. Semin Oncol 1997;24:686-95. [PubMed]
- Shipley WU, Tepper JE, Prout GR Jr, et al. Proton radiation as boost therapy for localized prostatic carcinoma. JAMA 1979;241:1912-5. [PubMed]
- Shipley WU, Verhey LJ, Munzenrider JE, et al. Advanced prostate cancer: the results of a randomized comparative trial of high dose irradiation boosting with conformal protons compared with conventional dose irradiation using photons alone. Int J Radiat Oncol Biol Phys 1995;32:3-12. [PubMed]
- Hanks GE. A question-filled future for dose escalation in prostate cancer. Int J Radiat Oncol Biol Phys 1995;32:267-9. [PubMed]
- Roach M 3rd. Dose escalated external beam radiotherapy versus neoadjuvant androgen deprivation therapy and conventional dose external beam radiotherapy for clinically localized prostate cancer: do we need both? Strahlenther Onkol 2007;183:26-8. [PubMed]
- Roach M 3rd, Bae K, Speight J, et al. Short-term neoadjuvant androgen deprivation therapy and external-beam radiotherapy for locally advanced prostate cancer: long-term results of RTOG 8610. J Clin Oncol 2008;26:585-91. [PubMed]
- Roach M 3rd. Current status of androgen suppression and radiotherapy for patients with prostate cancer. J Steroid Biochem Mol Biol 1999;69:239-45. [PubMed]
- Roach M 3rd. Neoadjuvant total androgen suppression and radiotherapy in the management of locally advanced prostate cancer. Semin Urol Oncol 1996;14:32-7; discussion 38. [PubMed]
- Guckenberger M, Meyer J, Baier K, et al. Distinct effects of rectum delineation methods in 3D-conformal vs. IMRT treatment planning of prostate cancer. Radiat Oncol 2006;1:34. [PubMed]
- Slater JD, Yonemoto LT, Rossi CJ Jr, et al. Conformal proton therapy for prostate carcinoma. Int J Radiat Oncol Biol Phys 1998;42:299-304. [PubMed]
- Slater JD, Rossi CJ Jr, Yonemoto LT, et al. Proton therapy for prostate cancer: the initial Loma Linda University experience. Int J Radiat Oncol Biol Phys 2004;59:348-52. [PubMed]
- Zietman AL, DeSilvio ML, Slater JD, et al. Comparison of conventional-dose vs high-dose conformal radiation therapy in clinically localized adenocarcinoma of the prostate: a randomized controlled trial. JAMA 2005;294:1233-9. [PubMed]
- Abramowitz MC, Li T, Buyyounouski MK, et al. The Phoenix definition of biochemical failure predicts for overall survival in patients with prostate cancer. Cancer 2008;112:55-60. [PubMed]
- Zietman AL, Bae K, Slater JD, et al. Randomized trial comparing conventional-dose with high-dose conformal radiation therapy in early-stage adenocarcinoma of the prostate: long-term results from proton radiation oncology group/american college of radiology 95-09. J Clin Oncol 2010;28:1106-11. [PubMed]
- Talcott JA, Rossi C, Shipley WU, et al. Patient-reported long-term outcomes after conventional and high-dose combined proton and photon radiation for early prostate cancer. JAMA 2010;303:1046-53. [PubMed]
- Mendenhall NP, Li Z, Hoppe BS, et al. Early outcomes from three prospective trials of image-guided proton therapy for prostate cancer. Int J Radiat Oncol Biol Phys 2012;82:213-21. [PubMed]
- Coen JJ, Bae K, Zietman AL, et al. Acute and late toxicity after dose escalation to 82 GyE using conformal proton radiation for localized prostate cancer: initial report of American College of Radiology Phase II study 03-12. Int J Radiat Oncol Biol Phys 2011;81:1005-9. [PubMed]
- Hara I, Murakami M, Kagawa K, et al. Experience with conformal proton therapy for early prostate cancer. Am J Clin Oncol 2004;27:323-7. [PubMed]
- Mayahara H, Murakami M, Kagawa K, et al. Acute morbidity of proton therapy for prostate cancer: the Hyogo Ion Beam Medical Center experience. Int J Radiat Oncol Biol Phys 2007;69:434-43. [PubMed]
- Sheets NC, Goldin GH, Meyer AM, et al. Intensity-modulated radiation therapy, proton therapy, or conformal radiation therapy and morbidity and disease control in localized prostate cancer. JAMA 2012;307:1611-20. [PubMed]
- Guckenberger M, Pohl F, Baier K, et al. Influence of rectum delineation (rectal volume vs. rectal wall) on IMRT treatment planning of the prostate. Strahlenther Onkol 2006;182:721-6. [PubMed]
- Goitein M, Cox JD. Should randomized clinical trials be required for proton radiotherapy? J Clin Oncol 2008;26:175-6. [PubMed]
- Fowler JF. The radiobiology of prostate cancer including new aspects of fractionated radiotherapy. Acta Oncol 2005;44:265-76. [PubMed]
- Munzenrider JE, Austin-Seymour M, Blitzer PJ, et al. Proton therapy at Harvard. Strahlentherapie 1985;161:756-63. [PubMed]
- Bush DA. Proton radiation therapy for lung cancer: is there enough evidence? Oncology (Williston Park) 2010;24:1052-7. [PubMed]
- Bush DA, Slater JD, Garberoglio C, et al. Partial breast irradiation delivered with proton beam: results of a phase II trial. Clin Breast Cancer 2011;11:241-5. [PubMed]
- Brenner DJ. Hypofractionation for prostate cancer radiotherapy--what are the issues? Int J Radiat Oncol Biol Phys 2003;57:912-4. [PubMed]
- Pollack A, Hanlon AL, Horwitz EM, et al. Dosimetry and preliminary acute toxicity in the first 100 men treated for prostate cancer on a randomized hypofractionation dose escalation trial. Int J Radiat Oncol Biol Phys 2006;64:518-26. [PubMed]
- Ritter M, Forman J, Kupelian P, et al. Hypofractionation for prostate cancer. Cancer J 2009;15:1-6. [PubMed]
- Spyropoulou D, Kardamakis D. Review of hypofractionated radiotherapy for prostate cancer. ISRN Oncol 2012;2012:410892.
- Fowler JF, Nahum AE, Orton CG. Point/Counterpoint. The best radiotherapy for the treatment of prostate cancer involves hypofractionation. Med Phys 2006;33:3081-4. [PubMed]
- Kuban DA, Tucker SL, Dong L, et al. Long-term results of the M. D. Anderson randomized dose-escalation trial for prostate cancer. Int J Radiat Oncol Biol Phys 2008;70:67-74. [PubMed]