TIME for biomarker-driven immunotherapy in non-small-cell lung cancer patients
More than 85% of lung cancer cases are classified as non-small-cell lung cancer (NSCLC), with predicted 5-year survival of 16% (1). However, after many years of dismal prognosis we are now experiencing a revolution in lung cancer treatment. Apart from targeted therapies, the potential of immunotherapy has created great excitement in the oncology community and has ushered in a new era of optimism. Cancer immunotherapy encompasses different approaches designed either to boost or restore immune functions. Therapeutic cancer vaccines are designed to stimulate the immune system of a cancer patient to act against tumor antigens (2). Sipuleucel-T, a vaccine designed to stimulate an immune response to prostatic acid phosphatase (PAP), was the first cancer vaccine approved for treatment of metastatic prostate cancer (3). Talimogene laherparepvec (T-VEC) is the first oncolytic virus therapy approved for inoperable metastatic melanoma. Unfortunately, other therapeutic cancer vaccines such as MAGE-A3 or tecemotide have not proved successful in the treatment of NSCLC (4,5). Checkpoint blockade therapy releases the ’brakes’ of the immune system and enhances the anti-tumor T-cell response (6) and in 2015 two such therapies, nivolumab (7,8) and pembrolizumab (9) were approved for the treatment of NSCLC.
Mucin 1 (MUC1) is a high molecular weight mucin-like transmembrane glycoprotein belonging to the mucin family (21 members) that is abnormally expressed in over 80% of all cancers. MUC1 functions as a tumor-associated antigen that induces CD8+ and CD4+ T-cell responses (10), as an intracellular signal transduction molecule and as a regulator of transcription of growth factors, similar to connective tissue growth factor (CTGF), platelet-derived growth factor A and B (PDGF-A and PDGF-B) (11) and multidrug resistance genes (MDR) (12). MUC1 directly associates with the epidermal growth factor receptor (EGFR) gene and translocates to the nucleus (11) (Figure 1).
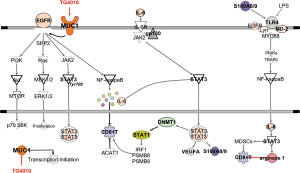
MUC1 has been defined as the second most promising target among 75 potential tumor associated antigens, but still there is no licensed product available against this target (13). Interesting results of disease stabilization have been described with the anti-MUC1 SP vaccine (ImMucin) in multiple myeloma patients (14,15). L-BLP25 (Stimuvax), developed by Merck, has now completed trials in NSCLC (16). TG4010 is a suspension of a modified vaccinia of Ankara that expresses the tumor antigen MUC1 and interleukin 2 (17). In a phase I clinical trial, TG4010 was shown to be safe and to have clinical activity (18). Two randomized studies have shown that the combination of TG4010 with chemotherapy in NSCLC patients is feasible and safe (19-21). The vaccine has also been tested in other types of tumors and the association between clinical activity of TG4010 and the cellular immune response against MUC1 has been demonstrated (22,23).
The TIME trial is a phase 2b/3 randomized, double-blind, placebo-controlled study evaluating TG4010 in combination with chemotherapy as first-line treatment of advanced NSCLC patients. Two hundred and twenty two patients from 45 centers in France, Belgium, the UK, Italy, Spain, Hungary, Poland, Israel and the USA were included. All patients had MUC1 expression by immunohistochemistry in at least 50% of the tumoral cells. The primary objective of the phase 2b part of the study was to analyze progression-free survival (PFS) and Quoix and colleagues demonstrated that adding TG4010 to first line chemotherapy improves PFS in comparison to placebo plus chemotherapy in advanced NSCLC patients (24). Overall, the TIME study demonstrated a significant benefit in PFS with the addition of TG4010 to first line chemotherapy. However, although the results were statistically significant, they were not notable, with an increase in PFS of only 5.9 months for patients in the TG4010 plus chemotherapy arm, compared to 5.1 months for the placebo plus chemotherapy arm. Interestingly, almost 50% of patients had unknown status of EGFR mutations at baseline. TG4010 was well tolerated and the trial is set to continue to phase 3.
Low baseline values of triple positive lymphocytes (TrPAL) for CD16, CD56 and CD69, corresponding to a phenotype of activated natural killer cells, were found to be predictive of TG4010 activity in combination with chemotherapy. In fact, the TrPAL test, developed as a companion diagnostic for TG4010, would be validated in the TIME trial. Validation was planned for if patients with baseline TrPAL values less or equal to the upper limit of normal (ULN) had a more than 95% probability of hazard ratio (HR) for PFS being less than 1. Also, and if patients with baseline TrPAL values greater than ULN had a more than 80% probability of HR for PFS of more than 1. The probability of HR being greater than 1 was 31.3%, and the primary endpoint was not met. From the clinical point of view, the most interesting results were in the 127 patients with non squamous histology and TrPAL values less than the third quartile (Q3). These patients had the highest benefit in terms of PFS and overall survival (OS) with the addition of TG4010 to chemotherapy (HR, 0.59, 95%CI 0.40–0.87; P=0.0033 and HR, 0.59 95%CI, 0.39–0.91; P=0.0072, respectively).
The pretreatment normal levels of activated natural killer cells (TrPAL) defined a subgroup of patients who derived significant benefit in several parameters including OS (20). But is there a reliable way to define the heterogeneous and plastic natural killer cells repertoire? There are two primary phenotypically defined subsets of natural killer cells. The majority has low-density expression of CD56 (CD56dim) and high levels of Fcγ receptor III (FcγRIII, CD16) (CD56dimCD16+), while others are CD56brightCD16dim or CD56brightCD16−. CD56dim natural killer cells predominate in the blood and are more cytotoxic than the CD56bright, which produce abundant cytokines following activation of monocytes but have low cytotoxicity (25). Therefore, natural killer cells should be treated as distinct CD56bright and CD56dim subsets, rather than as a homogenous population.
In a post-hoc exploratory analysis, programmed cell death 1 ligand 1 (PD-L1) expression was assessed by immunohistochemistry in pretreatment tumor specimens; both in tumoral cells and the immune infiltrate (24). Quoix and colleagues did not find improvement in PFS in the TG4010 group compared with placebo according to PD-L1 expression in tumoral cells. However, there was significant improvement in PFS in the TG4010 group compared with placebo according to PD-L1 expression in the immune infiltrate. Those patients with low PD-L1 expression in their immune infiltrate derived greater benefit from TG4010 (24) but whether these results are relevant is a matter of debate (26,27).
Immunotherapy trials targeting tumor antigens have yet to meet expectations. To better define patients who can benefit from immunotherapy, other factors such as tumor-infiltrating immune cells and gene signatures are now being evaluated. Hong at al. described a transcriptional signature related to innate anti-PD-1 resistance (28). CD8+ T cells play a significant role in antitumor immunity in many types of cancers (29) and it would be reasonable to assume that good prognosis is associated with CD8+ T cell infiltrate into the tumor, while accumulations of regulatory T cells (Treg) or myeloid-derived suppressor cells (MDSC) correlate with worse outcome. Signal transducer and activator of transcription 3 (STAT3) has been found to promote an immunosuppressive tumor microenvironment (30) (Figure 1). The S100A8 peptide belongs to the transcriptional network of STAT3 and decreases tumor infiltration of CD8+ T-cells, promoting a tumor growth-enhancing immune microenvironment through activation of the toll like receptor 4 (TLR4)/MD2 pathway (31) (Figure 1). Inhibition of acetyl-CoA acetyltransferase (ACAT1), a cholesterol esterification enzyme, significantly enhances the function and proliferation of CD8+ T-cells (32). The immunoproteosome (formed by proteasome subunits B; PSMB) generates peptides that bind to human leukocyte antigen (HLA) molecules, facilitating CD8+ T cell response. Reduced immunoproteosome expression has been linked to NSCLC with mesenchymal phenotype and reduced repertoire of HLA-bound peptides (33). STAT3 acts as an antagonist for STAT1, a key regulator of immunoproteosome and antigen presenting machinery (Figure 1) (33). STAT3 activates DNA methyltransferase 1 (DNMT1) which methylates the promoter region of interferon regulatory factor 1 (IRF1), PSMB8 and PSMB9 and HLA molecules that are crucial components of the antigen-presenting mechanism (33) (Figure 1).
A major impediment to cancer vaccines is that tumors with inherent resistance to T cell-mediated immunotherapy may never respond to therapies that target tumor antigens. Therefore, new strategies combining a MUC1 vaccine with antagonists of tumor-induced immune suppression are warranted.
Acknowledgments
Funding: None.
Footnote
Provenance and Peer Review: This article was commissioned and reviewed by the Section Editor Shao-Hua Cui (Department of Pulmonary Medicine, Shanghai Chest Hospital, Shanghai Jiao Tong University, Shanghai, China).
Conflicts of Interest: Both authors have completed the ICMJE uniform disclosure form (available at http://dx.doi.org/10.21037/tcr.2016.04.04). The authors have no conflicts of interest to declare.
Ethical Statement: The authors are accountable for all aspects of the work in ensuring that questions related to the accuracy or integrity of any part of the work are appropriately investigated and resolved.
Open Access Statement: This is an Open Access article distributed in accordance with the Creative Commons Attribution-NonCommercial-NoDerivs 4.0 International License (CC BY-NC-ND 4.0), which permits the non-commercial replication and distribution of the article with the strict proviso that no changes or edits are made and the original work is properly cited (including links to both the formal publication through the relevant DOI and the license). See: https://creativecommons.org/licenses/by-nc-nd/4.0/.
References
- Rosell R, Karachaliou N. Large-scale screening for somatic mutations in lung cancer. Lancet 2016; [Epub ahead of print]. [Crossref] [PubMed]
- Mellman I, Coukos G, Dranoff G. Cancer immunotherapy comes of age. Nature 2011;480:480-9. [Crossref] [PubMed]
- Kantoff PW, Higano CS, Shore ND, et al. Sipuleucel-T immunotherapy for castration-resistant prostate cancer. N Engl J Med 2010;363:411-22. [Crossref] [PubMed]
- Vansteenkiste JF, Cho B, Vanakesa T, et al. MAGRIT, a double-blind, randomized, placebo-controlled phase iii study to assess the efficacy of the recmage-a3 + as15 cancer immunotherapeutic as adjuvant therapy in patients with resected mage-a3-positive non-small cell lung cancer (NSCLC). Ann Oncol 2014;25:iv409.
- Carrizosa DR, Gold KA. New strategies in immunotherapy for non-small cell lung cancer. Transl Lung Cancer Res 2015;4:553-9. [PubMed]
- Pardoll DM. The blockade of immune checkpoints in cancer immunotherapy. Nat Rev Cancer 2012;12:252-64. [Crossref] [PubMed]
- Brahmer J, Reckamp KL, Baas P, et al. Nivolumab versus Docetaxel in Advanced Squamous-Cell Non-Small-Cell Lung Cancer. N Engl J Med 2015;373:123-35. [Crossref] [PubMed]
- Borghaei H, Paz-Ares L, Horn L, et al. Nivolumab versus Docetaxel in Advanced Nonsquamous Non-Small-Cell Lung Cancer. N Engl J Med 2015;373:1627-39. [Crossref] [PubMed]
- Garon EB, Rizvi NA, Hui R, et al. Pembrolizumab for the treatment of non-small-cell lung cancer. N Engl J Med 2015;372:2018-28. [Crossref] [PubMed]
- Roulois D, Grégoire M, Fonteneau JF. MUC1-specific cytotoxic T lymphocytes in cancer therapy: induction and challenge. Biomed Res Int 2013;2013:871936.
- Nath S, Mukherjee P. MUC1: a multifaceted oncoprotein with a key role in cancer progression. Trends Mol Med 2014;20:332-42. [Crossref] [PubMed]
- Nath S, Daneshvar K, Roy LD, et al. MUC1 induces drug resistance in pancreatic cancer cells via upregulation of multidrug resistance genes. Oncogenesis 2013;2:e51 [Crossref] [PubMed]
- Cheever MA, Allison JP, Ferris AS, et al. The prioritization of cancer antigens: a national cancer institute pilot project for the acceleration of translational research. Clin Cancer Res 2009;15:5323-37. [Crossref] [PubMed]
- Carmon L, Avivi I, Kovjazin R, et al. Phase I/II study exploring ImMucin, a pan-major histocompatibility complex, anti-MUC1 signal peptide vaccine, in multiple myeloma patients. Br J Haematol 2015;169:44-56. [Crossref] [PubMed]
- Kovjazin R, Horn G, Smorodinsky NI, et al. Cell surface-associated anti-MUC1-derived signal peptide antibodies: implications for cancer diagnostics and therapy. PLoS One 2014;9:e85400 [Crossref] [PubMed]
- Butts C, Murray N, Maksymiuk A, et al. Randomized phase IIB trial of BLP25 liposome vaccine in stage IIIB and IV non-small-cell lung cancer. J Clin Oncol 2005;23:6674-81. [Crossref] [PubMed]
- Limacher JM, Quoix E. TG4010: A therapeutic vaccine against MUC1 expressing tumors. Oncoimmunology 2012;1:791-2. [Crossref] [PubMed]
- Rochlitz C, Figlin R, Squiban P, et al. Phase I immunotherapy with a modified vaccinia virus (MVA) expressing human MUC1 as antigen-specific immunotherapy in patients with MUC1-positive advanced cancer. J Gene Med 2003;5:690-9. [Crossref] [PubMed]
- Ramlau R, Quoix E, Rolski J, et al. A phase II study of Tg4010 (Mva-Muc1-Il2) in association with chemotherapy in patients with stage III/IV Non-small cell lung cancer. J Thorac Oncol 2008;3:735-44. [Crossref] [PubMed]
- Quoix E, Ramlau R, Westeel V, et al. Therapeutic vaccination with TG4010 and first-line chemotherapy in advanced non-small-cell lung cancer: a controlled phase 2B trial. Lancet Oncol 2011;12:1125-33. [Crossref] [PubMed]
- Rotonda C, Anota A, Mercier M, et al. Impact of TG4010 vaccine on health-related quality of life in advanced non-small-cell lung cancer: results of a phase iib clinical trial. PLoS One 2015;10:e0132568 [Crossref] [PubMed]
- Oudard S, Rixe O, Beuselinck B, et al. A phase II study of the cancer vaccine TG4010 alone and in combination with cytokines in patients with metastatic renal clear-cell carcinoma: clinical and immunological findings. Cancer Immunol Immunother 2011;60:261-71. [Crossref] [PubMed]
- Dreicer R, Stadler WM, Ahmann FR, et al. MVA-MUC1-IL2 vaccine immunotherapy (TG4010) improves PSA doubling time in patients with prostate cancer with biochemical failure. Invest New Drugs 2009;27:379-86. [Crossref] [PubMed]
- Quoix E, Lena H, Losonczy G, et al. TG4010 immunotherapy and first-line chemotherapy for advanced non-small-cell lung cancer (TIME): results from the phase 2b part of a randomised, double-blind, placebo-controlled, phase 2b/3 trial. Lancet Oncol 2016;17:212-23. [Crossref] [PubMed]
- Cooper MA, Fehniger TA, Caligiuri MA. The biology of human natural killer-cell subsets. Trends Immunol 2001;22:633-40. [Crossref] [PubMed]
- Pennell NA. PD-L1 Testing and Lack of Benefit to Guide Treatment With Immune Checkpoint Inhibitors in Patients With Non-Small-Cell Lung Cancer. JAMA Oncol 2016. [Epub ahead of print].
- Lisberg A, Garon EB. The Value of PD-L1 Testing in Non-Small-Cell Lung Cancer. JAMA Oncol 2016. [Epub ahead of print].
- Hugo W, Zaretsky JM, Sun L, et al. Genomic and Transcriptomic Features of Response to Anti-PD-1 Therapy in Metastatic Melanoma. Cell 2016;165:35-44. [Crossref] [PubMed]
- Fridman WH, Pagès F, Sautès-Fridman C, et al. The immune contexture in human tumours: impact on clinical outcome. Nat Rev Cancer 2012;12:298-306. [Crossref] [PubMed]
- Jones LM, Broz ML, Ranger JJ, et al. STAT3 Establishes an Immunosuppressive Microenvironment during the Early Stages of Breast Carcinogenesis to Promote Tumor Growth and Metastasis. Cancer Res 2016;76:1416-28. [Crossref] [PubMed]
- Deguchi A, Tomita T, Ohto U, et al. Eritoran inhibits S100A8-mediated TLR4/MD-2 activation and tumor growth by changing the immune microenvironment. Oncogene 2016;35:1445-56. [Crossref] [PubMed]
- Yang W, Bai Y, Xiong Y, et al. Potentiating the antitumour response of CD8(+) T cells by modulating cholesterol metabolism. Nature 2016;531:651-5. [Crossref] [PubMed]
- Tripathi SC, Peters HL, Taguchi A, et al. Immunoproteasome deficiency is a feature of non-small cell lung cancer with a mesenchymal phenotype and is associated with a poor outcome. Proc Natl Acad Sci U S A 2016; [Epub ahead of print]. [Crossref] [PubMed]